Introduction
Enzyme Kinetics
Enzyme Classification
Transition State Theory
Enzyme Catalysis
Enzyme Active Sites and Enzyme-Substrate (ES) Complexes
Binding Energy
References
Enzymology is the study of enzymes and enzyme-catalyzed reactions. Enzymes are biological catalysts that accelerate the rate of biological reactions. They can also perform multiple reactions simultaneously, with the final distribution of reactants and products controlled by equilibrium properties.
Enzymes are predominantly proteins (although some are RNA-based) capable of acting under biological conditions to ensure a reaction occurs orders of magnitudes faster than chemically catalyzed counterparts. They are highly specific and regulated.
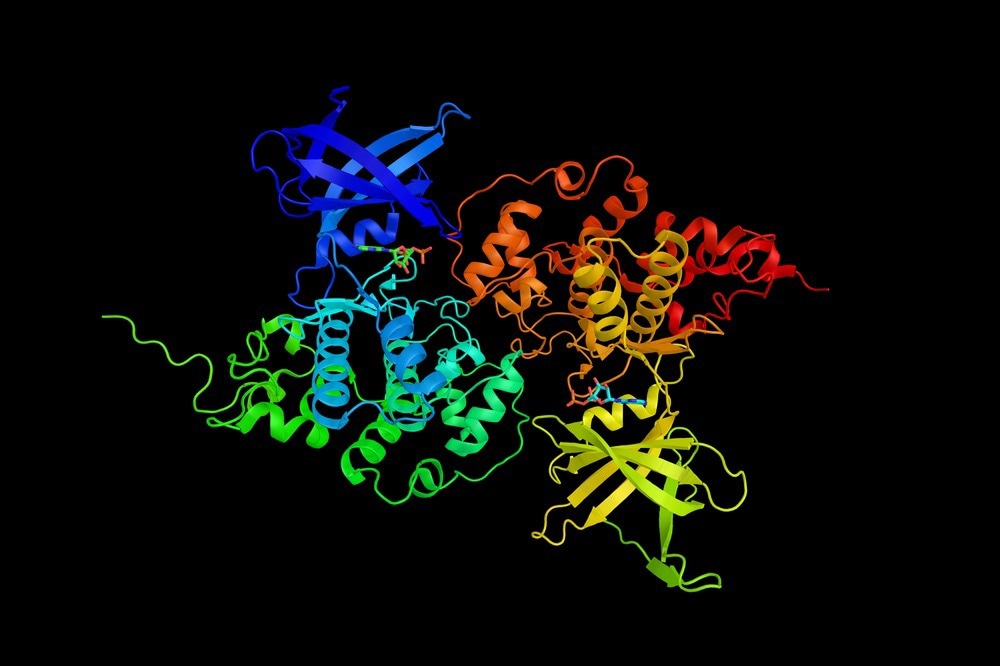
Image Credit: ibreakstock/Shutterstock.com
The study of enzymes and their functions includes:
- The molecular structure (primary, secondary, tertiary, and quaternary)
- Protein properties (isoelectric point, electrophoretic mobility, pH, temperature, stability, and spectroscopic properties)
- Kinetics: specificity, speed, and reversibility
- Thermodynamic properties: activation of free energy and entropy
- Active site: including the number of available sites, the molecular nature of the site, and the mechanism of catalysis
- Biological properties (location in the cell/ compartment(s), isoenzyme forms, and metabolic implications of the reaction carried out)
Enzyme Kinetics
A substrate is a reactant in an enzyme-catalyzed reaction. The rate enhancement achieved by an enzyme is often several orders of magnitude greater than the non-enzymatic rate.
Unique interactions at the molecular level between the enzyme and the substrate can account for this enhancement. An enzyme-substrate complex is formed in part of the protein molecule called the active site. Active sites contain several unique features left to enable optimal positioning of the relevant residues in both the substrate and enzyme to minimize the thermodynamic barrier.
Another key characteristic of enzymes is their ability to specifically bind substrates. Enzymes can discriminate between very closely related molecules; three structural determination methods, the enzyme-substrate complex, and its analog can be directly observed.
Enzyme Classification
Enzymes are classified according to the types of reactions they can catalyze:
- Oxidoreductases carry out redox reactions
- Transferases transfer functional groups
- Lyase: utilize group elimination to form double bonds
- Isomerases: carry out isomerization
- Ligases: join molecules with ATP or other nucleotide triphosphate hydrolysis
Enzymes thermodynamics kinetics and enzymology Gibbs free energy (G) is found to be the most useful function for enzyme ology. The free energy change, ΔG, for a process determines whether or not the process is spontaneous under a specific set of conditions. Equilibrium conditions are governed by the standard free energy change ΔG° (ΔG, with a superscript o, is the free energy change for a reaction, with everything in the standard states).
When ΔG < 0, the process is spontaneous according to thermodynamics. However, even if ΔG < 0, the reaction may not occur as the rate of reaction may be too slow to be observed, i.e., while the reaction may be thermodynamically feasible, it is not kinetically feasible.
When ΔG = 0, an equilibrium dynamic is present, and there is no net change in composition. Here G is at its minimum value. If ΔG > 0, a process cannot occur spontaneously; for this reaction to occur, it must be coupled with another process for which ΔG < 0 and is of a greater magnitude so that the net ΔG for both of these processes, when summed, is negative.
The relationship between free energy and the equilibrium constant is ΔG°′ = −RT ln K′, where ΔG°′ is the biochemical standard free energy change for the reaction, and K′ is the equilibrium constant.
Enzymes do not alter the magnitude of ΔG, the rate at which a reaction approaches equilibrium by selectively lowering the theoretical free energy required to reach the transition state (ΔG‡) after the enzyme-substrate complex has formed. This is related to the transition state stabilization, which denotes the enzyme's preferential binding for the transition state (ES‡) complex.
Transition State Theory
The transition state, ES‡, is metastable and, by definition, a transition state cannot be isolated. The transition state shares some features of both the reactants and products. In this state, some bonds in the substrate are broken, while product bonds are partially formed. Enzymes can cause a rate enhancement by reducing the value of ΔG ‡, the free energy of activation.
The velocity (rate) of the reaction is proportional to the reactant's concentration and the ratio's exponential (−ΔG‡/RT). In practice, at 25°C, an enzyme can achieve a 10-fold rate enhancement for each 5.71 kJ/mol (1.36 kcal/mol).

Image Credit: Design_Cells/Shutterstock.com
Enzyme Catalysis
The mechanism that defines a reaction catalyzed by an enzyme may occur via a sequence of distinct steps and several transition states. Proteins are considered to be optimized for the role of an enzyme due to their structural and chemical capabilities. There is a unifying mechanistic set of principles that can be applied to a variety of diverse enzymes under the reaction they catalyze. Therefore, a comprehensive understanding of organic chemistry is essential because there are recurring functional groups such as carbonyl compounds and carboxylic acid derivatives form the basis of enzyme-catalyzed reactions.
Moreover, the twenty amino acids have great chemical diversity, which enables proteins to catalyze reactions via several chemical mechanisms: general acid/general base catalysis, covalent catalysis, and metal ion catalysis.
Many reactions in organic chemistry occur via general acid or general base mechanisms or involve a nucleophilic attack. This occurs most notably in covalent catalysis. In the catalytic mechanism, groups such as COOH and ImH+ (protonated imidazole) operate as proton donors (or general acids), while groups such as NH2, COO−, and neutral imidazole function as proton acceptors (general bases). The side chains which contain catalytically important residues include nucleophilic (electron-pair donors) groups such as the -OH and -SH groups of Ser and Cys, respectively.
Enzyme Active Sites and Enzyme-Substrate (ES) Complexes
The active site of an enzyme is a three-dimensional pocket that forms by the arrangement in the space of different amino acid residues. There is a great difference between primary and tertiary structures; amino acids separated by large distances from the primary sequence interact in the tertiary structure reaction. Spectroscopic methods like fluorescence spectroscopy have been used to determine what changes occur when substrates are added. X-ray crystallography of enzyme-substrate or enzyme-substrate analog complexes has since produced detailed examples of how enzymes recognize and bind substrates.
Many enzymes catalyzed reactions involve more than one substrate, which leads to the important mechanistic principle; of proximity and orientation effects. If two substrates are brought together in proximity, they must be brought together to allow the best orientation for a reaction. The directionality of the chemistry of a reaction influences this. An enzyme does not only bind substrates close together (proximity effect) within an active site but also in an optimized and precise orientation that favors the reaction being catalyzed.
Binding Energy
Binding energy is the energy change that occurs when the enzyme-substrate complex is formed. To illustrate binding energy, lysozyme can be used. The lysozyme has a large substrate that can make energetically favorable contacts. However, the Liza zyme does not have a great affinity for its substrate/ find it strongly. It is structured to hydrolyze optimally, not bind as tightly as possible.
A portion of the points of contact the enzyme makes with the substrate can distort part of it to resemble the transition state. In this way, the enzyme selectively lowers the reaction transition state energy. Subsequently, lysozyme binds the transition state better than it does the substrate. Therefore, some potential binding energy used to form the enzyme-substrate complex is used to bind a higher energy form of the substrate more effectively.
At present, enzyme ology is being studied using computation. This article gives a brief overview of the study of the subject; however multidisciplinary research field which integrates not only biochemistry, but molecular biology, genetics, biophysics, microbiology, and chemistry. To investigate enzymology, there is there on the development of activity assays, the overexpression and purification of enzymes, characterization of steady-state kinetics, as well as an initial basic structural characterization of the enzyme itself 2–which may include determining the structure of the subunits, the molecular mass, the presence of prosthetic groups and cofactors, and any post-translational modifications which may affect the rate of reaction and enzyme behavior. Overall, the literature addressing catalytic enzyme mechanisms is enormous – and continues to grow.
References:
- Donald Voet, Judith G. Voet, Charlotte W. Pratt. Fundamentals of Biochemistry, 4th ed.; Chs.11 & 12.
- Silverman RB. The Organic Chemistry of Enzyme-Catalyzed Reactions (Revised ed., 2002, Academic Press).
Further Reading