Fluorescent nanodiamonds (FNDs) have unique characteristics, including excellent biological compatibility, limitless photostability, no photoblinking, extended fluorescence lifespan (>10 ns), and ease of biofunctionalization. These qualities make FNDs a favorable choice for imaging compared to quantum dots, organic dyes, and fluorescent polymer beads.
FND fluorescence comes from nitrogen-based color centers. Their stability is due to being securely embedded in the lattice and shielded from external factors.
FNDs are used for various purposes, such as long-term cell imaging without background interference, tracking cell lineage, flow cytometry, high-resolution imaging, combined microscopy techniques, labeling rare cellular components, and guiding surgical procedures based on imaging.
Apart from its distinct fluorescence, the negatively charged nitrogen-vacancy color center in FNDs is spin-active. This allows FNDs to be modulated using external factors like electromagnetic fields, temperature, and strain.
The unique abilities of FNDs make them exceptional quantum nanosensor materials, enabling precise measurement of temperature changes within an intracellular environment.
Characteristics of fluorescent nanodiamonds: Color, brightness, and size
The light emitted by the main color centers in FNDs covers the visible spectrum, and the specific emission wavelength is adjusted by changing the nitrogen (N) and vacancy (V) content. For instance, FNDs containing NV centers emit red/near-infrared light, while those with NVN centers emit green light. FNDs with N3 centers emit blue light.
Among the FNDs with different color centers, those with NV and NVN centers are the most extensively studied.
The fluorescence intensity in FNDs is influenced by their size, ranging from 10 nm to 1 μm, because more centers per particle become available with increasing particle volume. Studies have shown that an 80 nm FND containing 3 ppm NV- centers, as measured by electron paramagnetic resonance, emits light approximately 10 times brighter than a single Atto 532 dye molecule.
FNDs, being carbon-based, undergo surface modification through organic functional group changes. Major chemical functionalities available for FNDs are carboxylic acids (-COOH), hydroxyls (-OH) groups, and amines (-NH2).
These functionalities allow for the conjugation of ligands using a range of conventional organic chemistries, such as amidation, esterification, and silanization. Among different surface-modified FNDs, carboxylated FNDs are especially versatile, serving as a crucial starting point for subsequent modifications essential in biological applications.
Nanodiamonds scatter light effectively, making them valuable for guiding and reducing light intensity. In terms of appearance, solutions containing large nanodiamond particles look milky-white until about 50 nm. Beyond 50 nm, the solution begins to turn transparent with a brown/amber hue (see Figure 1).
The clarity of light transmitted is an indication of particle size and, as such, is useful for monitoring colloidal stability during particle functionalization. If nanodiamonds look hazy or gray after functionalization or alteration, this could be an indication that they might have aggregated. Often, this aggregation is temporary and can be fixed by placing the particles in a solvent that suits their surface chemistry, either hydrophilic or hydrophobic.
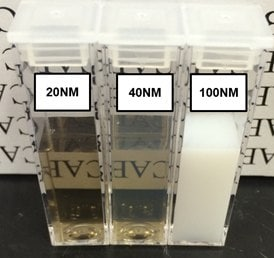
Figure 1. Suspensions of 20, 40, and 100 nm FND particles in deionized water. Extensive light scattering of large particles causes the 100 nm suspension to appear milky-white in color. The slight reduction in optical clarity of the sample containing 40 nm particles as compared to the one with 20 nm particles is indicative of greater light scattering from larger particles. Suspensions adopt a brown/amber color when sizes are small enough that light scattering is reduced. In all these suspensions the concentration of FNDs is approximately 1 mg/mL. Image Credit: Merck
Although the majority of commercial FND particles are marketed on a weight per unit volume (w/v) basis, it is still important to understand their particle number concentration (i.e., particles per unit volume) for further surface modification.
When particle size decreases, but the mass remains constant, the concentration of particles significantly rises. This increases the number of potential reactive sites and reduces space between particles, increasing the likelihood of aggregation. Therefore, adjusting the particle number concentration is necessary for efficient functionalization.
The particle number concentration (C (M)) can easily be determined by inputting the particle diameter d (nm) and weight/volume concentration (x in mg/mL) derived from diamond density, mass per particle, and Avogadro’s constant:
 |
(1) |
The number of surface sites available in a reaction (N) involves the areal density of surface COOH groups (ρ, roughly 0.5-1 group/nm2), volume of particle solution used (V), and particle surface area (d):
 |
(2) |
Conjugation protocols for nanodiamonds
a) Streptavidin functionalization
Carboxy polyethylene glycol ND (ND-PEG-COOH) is a useful starting point for streptavidin functionalization.
While the reaction can be performed in water, doing so in DMF boosts the stability of NDs, improves NHS-sulfo activation, and minimizes aggregation. It is important to consider the number of available reactive sites while determining specific reaction conditions because this varies with size.
- Centrifuge ND particles in water at the lowest RCF are required to form a pellet.
- Suspend the pellet in DMF at a concentration of <50 nM (usually <1 mg/mL) and bath sonicate for 5 minutes. Particles should resuspend readily.
- Add NHS-sulfo and EDC in ~300x molar excess to the number of reactive sites while adding NHS-sulfo first. For example, using expression 1 & 2 the amount of NHS-sulfo and EDC for 50 nm particle is estimated to be 140 nmol/mg. It should be noted that in most cases, 1 mg of EDC/NHS is more than sufficient unless aggregation becomes a problem. In the instances where aggregation becomes a concern, the amounts of EDC/NHS should be lowered.
- Briefly vortex the solution from step 3, allowing it to react for 30 minutes with occasional vortexing and bath sonication. The solution might become hazy with slight aggregation.
- Wash particles in DMF by pelleting them at a low RCF (<1000× g should be enough) and re-suspending them in DMF.
- Pellet at low RCF and resuspend in water (<50 nM, generally <1 mg/mL) to thoroughly break the pellet. For optimum stability, conjugation should be performed in DI or a low ionic strength (<10 mM) buffer at this step.
- Add an excess of streptavidin. Adjust the amount based on the desired coverage and let the reaction proceed for 2-4 hours. Note that 100x streptavidin compared to particle number concentration is a good starting point. However, reduce it if aggregation occurs. The surplus is crucial to prevent streptavidin interparticle bridging.
- Rinse the particles at least twice with the preferred end buffer to eliminate excess streptavidin. If centrifuging, the remaining reactive groups on the ND surface may be quenched via the addition of a small molecule containing an amine (tris or glycine) to avoid aggregation from pelleting. Alternatively, at this stage, dialyzing particles with a large pore size (>150 kDa MWCO) membrane can prevent centrifugation-based aggregation. For increased stability, final particles can be resuspended in 0.1% BSA in PBS.
- FNDs should be kept at 4 °C (not frozen) after being functionalized with streptavidin. Functionalized NDs that have been appropriately stored should be active for several months
b) Biotinylation
Biotinylation of NDs is simple; it can be done by reacting NHS-conjugated PEG-biotin (Aldrich Prod. No. 670049) with aminated NDs (ND-NH2). Alternatively, aminated NDs with a PEG coating can also be conjugated directly to Biotin-NHS.
- ND-NH2 is centrifuged out at low RCF (e.g. 5000 rcf) from water and resuspended in DMF (or another suitable NHS conjugation buffer).
- NHS-PEG-Biotin is applied to reactive sites (1–10×) in the necessary molar excess and reacted overnight.
- Particles should be washed twice with DMF or water before being suspended in the selected final buffer.
- Particles should be kept at 4 °C (not frozen). Functionalized NDs that have been appropriately stored should remain active for several months.
c) Coupling biotinylated/streptavidin functionalized nanodiamonds
Coupling between biotinylated and streptavidin functionalized moieties is simple and requires only a brief period of incubation (e.g., 30 minutes). Coupling of larger particles or inter-particle coupling may take longer to conjugate due to reduced translational and rotational freedom induced by the particle size and statistical chances of binding.
When planning incubation periods, it is critical to consider and modify the multivalency of either streptavidin or biotinylated elements. Non-specific binding can be prevented by adding blocking agents (such as BSA, detergent, or glycerol) without affecting streptavidin/biotin activity.
In conclusion, fluorescent nanoparticles (FNDs) are suitable fluorescent platforms for biomedical applications that need sustained and/or strong lighting. FND conjugation is similar to that of traditional organic-based molecular fluorochromes, and their per-particle brightness makes them ideal for marking low-level expressed targets.
Their realization in the laboratory can assist researchers in increasing their capabilities beyond those of classic organic dyes.
To find out more, request the “Nanomaterial Bioconjugation Techniques” handbook, which includes step-by-step techniques for surface modification and bioconjugation of inorganic nanomaterials for diagnostic purposes.
Acknowledgments
The NHLBI, Department of Health and Human Services, financed the work under Contract No. HHSN268201500010C.
Applications
FNDs can be used as an in vivo nanoprobe for bioimaging, cell labeling, and cell tracking.
Source: Merck
. |
. |
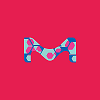 |
Fluorescent nanodiamond Nitrogen vacancy 2.5 ppm NV centers, 70 nm avg. part. size, 1 mg/mL in deionized water Nanodiamonds |
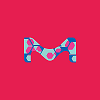 |
Fluorescent nanodiamond Nitrogen vacancy 3 ppm NV centers, 120 nm avg. part. size, 1 mg/mL in deionized water Nanodiamonds |
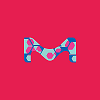 |
Fluorescent nanodiamond Nitrogen vacancy 4 NV/particle, 35 nm avg. part. size (DLS), 1 mg/mL in deionized water, carboxylated Nanodiamond |
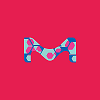 |
Fluorescent nanodiamond Nitrogen vacancy 3 ppm NV centers, 100 nm avg. part. size, 1 mg/mL in deionized water Nanodiamonds |
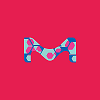 |
Fluorescent nanodiamond Nitrogen vacancy 1.5 ppm NV centers, 100 nm avg. part. size, 1 mg/mL in deionized water Nanodiamonds |
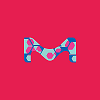 |
Fluorescent nanodiamond Nitrogen vacancy 3 ppm, 100 nm avg. part. size (APS), aminated powder Nanodiamond |
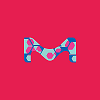 |
Fluorescent nanodiamond Nitrogen vacancy 3 ppm NV centers, 90 nm avg. part. size, 1 mg/mL in deionized water Nanodiamonds |
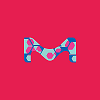 |
Fluorescent nanodiamond Nitrogen vacancy 900 NV/particle, 100 nm avg. part. size (DLS), 1 mg/mL in deionized water, hydroxylated Nanodiamond |
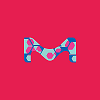 |
Fluorescent nanodiamond Nitrogen vacancy 60 NV/particle, 70 nm avg. part. size (DLS), 1 mg/mL in deionized water Nanodiamond |
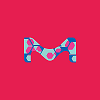 |
Fluorescent nanodiamond Nitrogen vacancy 3 ppm NV centers, 140 nm avg. part. size, carboxylic acid functionalized, PEG 3000 coated, 1 mg/mL in deionized water Nanodiamonds |
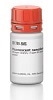 |
Fluorescent nanodiamond Nitrogen vacancy 3 ppm NV centers, 140 nm avg. part. size, amine functionalized, powder Nanodiamonds |
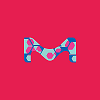 |
Fluorescent nanodiamond Nitrogen vacancy 3 ppm, 140 nm avg. part. size, amine functionalized, PEG 3000 coated, 1 mg/mL in deionized water Nanodiamonds |
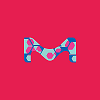 |
Fluorescent nanodiamond Nitrogen vacancy 3 ppm, 140 nm avg. part. size, hydroxyl functionalized, PEG 3000 coated, 1 mg/mL in deionized water Nanodiamonds |
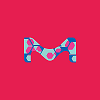 |
Fluorescent nanodiamond Nitrogen vacancy 3 ppm NV centers, 140 nm avg. part. size, biotin functionalized, PEG 3000 coated, 1 mg/mL in deionized water Nanodiamonds |