Alzheimer's disease, often abbreviated as AD, represents a multifaceted neurological condition that exerts a substantial influence on the elderly demographic. Its onset involves pathological transformations within the brains of affected individuals, preceding any visible clinical symptoms.
These changes encompass the build-up of amyloid-β (Aβ) toxins, the development of neuroprotofibrillary tangles (NFTs) due to hyperphosphorylated tau proteins, and the neurodegenerative effects stemming from the release of neurotoxins and inflammatory factors.
Molecular biomarkers, such as APP (Amyloid precursor protein), Tau, BACE1 (Beta-site APP-cleaving enzyme 1), ApoE (Apolipoprotein E), NGF (Nerve Growth Factor), BDNF (Brain-Derived Neurotrophic Factor), GLP1R (Glucagon-Like Peptide-1 Receptor), GSK3B (Glycogen Synthase Kinase 3 Beta), CASP9 (Caspase-9), CLU (Clusterin), AGER (Advanced Glycation End Product Receptor), DPYSL2 (Dihydropyrimidinase Like 2), and PNMT (phenylethanolamine-N-methyl-transferase), are pivotal in contributing to its pathogenesis.
Diverse research endeavors harness these biomarkers, probing their implications in neuroprotection, tau uptake, and neurite growth. These biomarkers play essential physiological roles, but when dysregulated, they can also exacerbate pathological conditions.1-6
The journey from research to clinical breakthroughs is a formidable one, underscored by continuous trials focused on innovative approaches to combat Alzheimer’s disease, targeting Aβ, tau, neuroinflammation, and synaptic preservation.1,3 It is worth noting the emergence of FDA-approved anti-Aβ antibodies like aducanumab and lecanemab.7,8
In the research domain, recombinant proteins like Aβ, tau, GLP1R, and ApoE are instrumental in advancing drug discovery and unraveling target interactions.4,9–12 Leading the charge in Alzheimer's research, Sino Biological leads the way by supplying recombinant Alzheimer's biomarkers and contributing invaluable insights for disease diagnosis and the development of groundbreaking therapeutics.
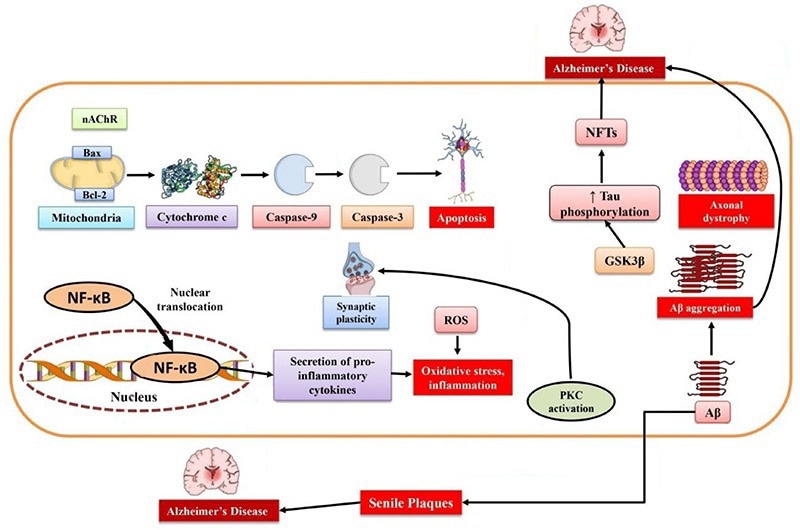
Schematic of Alzheimer’s Disease Pathophysiology. Image Credit: doi.org/10.3390/md19050251
Physiological and pathological roles
A cluster of biomarkers assumes central roles in both normal physiological functions and the emergence of pathological states. NGF, for instance, plays a crucial role in promoting the growth and upkeep of nerve cells, but when its regulation falters, it becomes linked to neurodegenerative ailments like Alzheimer’s.13
CASP9 is indispensable for orchestrating programmed cell death (apoptosis) and maintaining tissue equilibrium, yet its activation in the Alzheimer’s disease-afflicted brain could precipitate the formation of neuroprotofibrillary tangles (NFTs).14
Similarly, CLU serves diverse physiological functions, ranging from lipid transport to cell differentiation, but heightened levels are evident in neurodegenerative conditions such as Alzheimer’s disease.15
AGER contributes to normal tissue development but, under pathological conditions, it can incite inflammation and oxidative stress. DPYSL2, a critical player in neuronal development, might also have links to neurodegenerative disorders.16,17
PNMT is pivotal for synthesizing stress-related hormones, while BDNF supports neuronal survival and adaptability.18 GSK3B, responsible for regulating various cellular functions, becomes problematic when dysregulated, potentially leading to neurodegenerative and mood disorders.19,20
BACE1, another biomarker, is implicated in the production of Aβ in Alzheimer's disease, rendering it a focal point for therapeutic strategies in AD.21 GLP1R agonists have demonstrated their potential in countering neuroinflammation, reducing oxidative stress, and promoting neurotrophic effects, positioning them as potential candidates for Alzheimer's disease treatment.12,22
From bench to bedside
The journey from laboratory discoveries to achieving clinical breakthroughs demands meticulous examination and validation. Within the realm of clinical trials assessing innovative Alzheimer's disease treatments, substantial focus has been directed toward the reduction of Aβ accumulation and aggregation.
Prominent examples of success in this pursuit include the FDA approvals of anti-Aβ antibodies, such as aducanumab in 2021 and lecanemab in 2023, marking significant milestones.7,8
ApoE, particularly the ApoE ε4 allele, stands as a well-established genetic risk factor for Alzheimer’s disease. Approaches aimed at modulating ApoE expression and the pathways associated with lipid metabolism hold promise in reducing Aβ deposition and enhancing Aβ clearance.10
Alternative strategies involve the utilization of BACE inhibitors to decrease the production of Aβ and γ-secretase modulators to impact Aβ cleavage processes.21 Another pivotal aspect of Alzheimer's disease pathology is the aggregation of tau proteins.
Ongoing research is focused on inhibiting tau kinases and stabilizing microtubules to prevent the formation of tau tangles.11 It is of utmost importance to maintain synaptic function and connectivity when addressing Alzheimer's disease treatment.
Neurotrophic factors like NGF and BDNF are under investigation as potential means to bolster neuronal survival and promote synaptic plasticity.13,18
GLP-1 receptor agonists, initially sanctioned for managing type 2 diabetes and obesity, are being explored for their potential in Alzheimer’s disease treatment through the mitigation of neuroinflammation.22
Application in research
To advance drug discovery and gain insights into target interactions, the availability of high-quality reagents is imperative.
Recombinant proteins, such as Aβ peptides, tau protein, and ApoE variants, play a crucial role in facilitating mechanistic studies and preclinical evaluations, thereby contributing to the development of innovative therapies for Alzheimer's disease.
In their research, Gao et al. examined the interaction between GSK-3β and PKG using the human GSK-3β protein. They determined that the neuroprotective effects of icariside II were linked to its interference with the PKG/GSK-3β/autophagy axis.20
Similarly, Endicott et al. conducted experiments involving the treatment of lysosomes with Tau protein and protease inhibitors to investigate chaperone-mediated autophagy (CMA) substrate uptake within isolated lysosomes.
Their findings revealed enhanced uptake of Tau protein and reduced GFAP phosphorylation in liver lysosomes from mice treated with buparlisib or pictilisib.23
Sperling and colleagues conducted a study in which they examined the impact of AChE binding to laminin-1 on AChE activity, resulting in increased neurite growth in culture. However, they could only detect cell-associated recombinant AChE in approximately 0.5% of the cells, primarily within the cell body.6
In a separate investigation, Zhang and his team explored the interaction between their graphene oxide (GO) nanoparticles and purified APP or BACE1 protein (provided by Sino Biological). Their findings indicated that GO treatment reduced Aβ levels and enhanced memory in mice.24
Vojdani and colleagues employed recombinant β-NGF and BDNF proteins (obtained from Sino Biological) in their study to examine the immune reactivity of Anti-Aβ-42 peptide with tissue antigens that could potentially play a role in neurodegenerative disorders. They observed that the antibody exhibited significant reactivity with β-NGF and BDNF.25
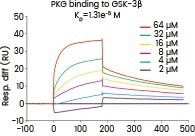
A surface plasmon resonance assay was conducted to assess the binding affinity of PKG to GSK-3β (Cat#: 10044-H07B, Sino Biological). Various concentrations of PKG were combined with GSK-3β, and the resulting binding was detected. Image Credit: Doi.org/10.1111/bph.14912
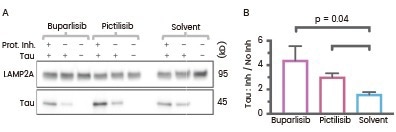
Liver lysosomes from buparlisib or pictilisib treated mice have increased CMA substrate Tau (Cat#: 10058-H07E, Sino Biological) uptake and decreased GFAP phosphorylation. Image Credit: Doi: 10.1083/jcb.202001031.
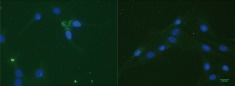
Immunostaining with an anti (His)6Tag antibody (green) of R28 cells treated with (His)6Tag E6-AChE (Cat#: 50543-M08H, Sino Biological). Cell nuclei are stained with DAPI (blue). Image Credit: DOI:10.1371/journal.pone.0036683.
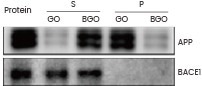
Assessment of the interaction between GO/BGO and APP, with Western blot outcomes for APP and BACE1 proteins (Sino Biological). S and P are the supernatant and the pellet, respectively, derived from the precipitation. Image Credit: doi.org/10.7150/thno.50616.
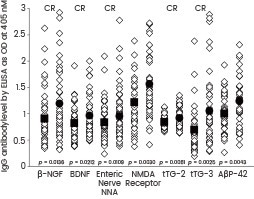
Scattergrams of antibody levels expressed by ELISA ODs in nondemented controls and AD sera. IgG antibodies against various proteins and peptides, including β-NGF and BDNF (Sino Biological), were involved in AD. Image Credit: Doi: 10.1155/2018/1672568.
Featured recombinant proteins for AD drug targets
- HPLC-verified
- Validated activity
- Broad coverage of species and tags
- Highly cited in reputable journals
Human GLP1R protein (His & AVI tag)
(Cat#: 13944-H49H-B)
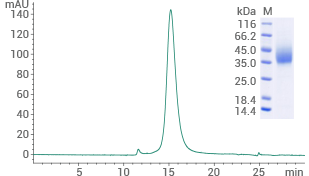
Purity ≥ 95% (SEC-HPLC). Image Credit: Sino Biological US Inc
Human BACE1 protein (His tag)
(Cat#: 10064-H08H)
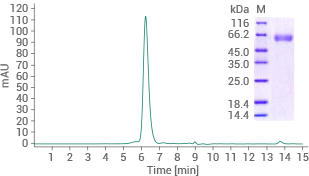
Purity ≥ 95% (SEC-HPLC). Image Credit: Sino Biological US Inc
Human RAGE protein (No tag)
(Cat#: 11629-HCCH)
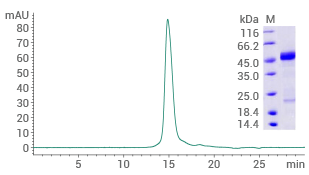
Purity ≥ 90% (SEC-HPLC). Image Credit: Sino Biological US Inc
Human GSK3B protein (His tag)
(Cat#: 10044-H07B)
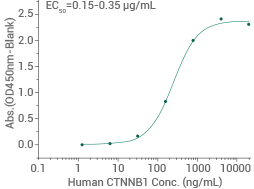
Activity-validated: Immobilized His-GSK3B can bind to biotinylated human HG3C-CTNNB1 (Cat#: 11279-H20B). Image Credit: Sino Biological US Inc
Human NGF protein (No tag)
(Cat#: 11050-HNAC)
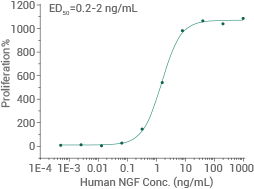
Cell proliferation assay using TF-1 human erythroleukemic cells. Image Credit: Sino Biological US Inc
Human alpha-synuclein protein (H50Q)
(Cat#: 12093-HNAE1)
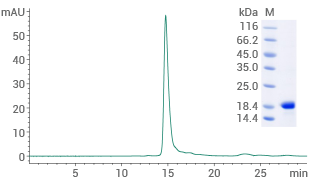
Purity ≥ 95% (SEC-HPLC). Image Credit: Sino Biological US Inc
More recombinant Alzheimer’s drug targets
Source: Sino Biological US Inc
Product |
Cat# |
Tag |
Citations |
Mouse NGF Protein |
50385-MNAC |
No Tag |
4 Citations |
Human GLP1R Protein |
13944-H02H |
hFc Tag |
2 Citations |
Human Tau Protein |
10058-H07E |
His Tag |
1 Citation |
Mouse GSK3B Protein |
50650-M07B |
His Tag |
1 Citation |
Human APP Protein |
10703-H02H |
hFc Tag |
|
Human Clusterin Protein |
11297-H08H |
His Tag |
|
Human BACE1 Protein |
10064-H02H |
hFc Tag |
|
Human ApoE Protein (E3) |
10817-H30E-B |
His & Trx Tag, Biotinylated |
|
Mouse BDNF Protein |
50240-M08H |
His Tag |
|
Mouse RAGE Protein |
50489-M08H |
His Tag |
|
Mouse AChE Protein |
50543-M08H |
His Tag |
|
Human Beta-amyloid 40 Protein |
10703-H20E1 |
His & GST Tag |
|
Featured antibodies against AD targets
Anti-BACE1 antibody, Rabbit mAb
(Cat#: 10064-R027)
Applications: FCM, ICC/IF
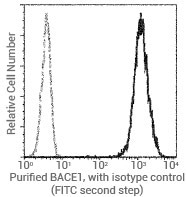
Flow cytometric analysis of human BACE1 expression on Jurkat cells. Image Credit: Sino Biological US Inc
Anti-RAGE antibody, Mouse mAb
(Cat#: 11629-MM02)
Applications: IHC-P
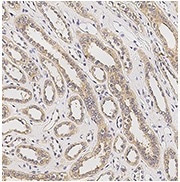
Immunochemical staining of human AGER in human kidney. Image Credit: Sino Biological US Inc
Anti-Clusterin antibody, Mouse mAb
(Cat#: 11297-MM01)
Applications: WB, ELISA, FCM, ICC/IF
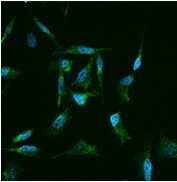
Immunofluorescence staining of human CLU in Hela cells. Image Credit: Sino Biological US Inc
Anti-Caspase-9 antibody, Rabbit pAb
(Cat#: 310364-T08)
Applications: IHC-P
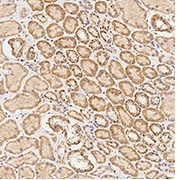
Immunochemical staining of human Caspase-9 in human kidney. Image Credit: Sino Biological US Inc
Anti-ApoE antibody, Rabbit pAb
(Cat#: 10817-RP02)
Applications: ELISA, IHC-P
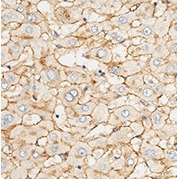
Immunochemical staining of human APOE in human liver. Image Credit: Sino Biological US Inc
Anti-DPYSL2 antibody, Rabbit pAb
(Cat#: 203068-T38)
Applications: WB, ICC/IF, IP
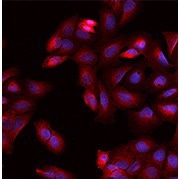
Immunofluorescence staining of DPYSL2 in U2OS cells. Image Credit: Sino Biological US Inc
More antibodies against AD targets
Source: Sino Biological US Inc
Product |
Cat# |
Applications |
NGF/NGFB Neutralizing Antibody |
11050-R711 |
Neutralization |
Anti-Caspase-9 Antibody, Rabbit pAb |
102185-T38 |
WB, ICC/IF, IP |
Anti-BACE1 (FITC) Antibody, Rabbit mAb |
10064-R027-F |
FCM |
Anti-Clusterin Antibody, Rabbit mAb |
11297-R210 |
IHC-P |
Anti-RAGE Antibody, Rabbit pAb |
11629-T24 |
ELISA, IHC-P |
Anti-DPYSL2 Antibody, Rabbit pAb |
109663-T08 |
IHC-P |
Anti-PNMT Antibody, Rabbit pAb |
204179-T36 |
WB, IP |
Anti-GSK3B Antibody, Rabbit pAb |
50650-T38 |
WB, ICC/IF, IP |
Anti-ApoE Antibody, Rabbit pAb |
10817-RP01 |
ELISA |
Anti-alpha-Synuclein Antibody, Rabbit pAb |
12093-T62 |
WB, ELISA, IHC-P, ICC/IF, IP |
Learn More about Reagents
References
- Husna Ibrahim, N. et al. Pharmacotherapy of Alzheimer’s Disease: Seeking Clarity in a Time of Uncertainty. Frontiers in Pharmacology vol. 11 Preprint at https://doi.org/10.3389/fphar.2020.00261 (2020).
- Hansson, O. et al. The Alzheimer’s Association appropriate use recommendations for blood biomarkers in Alzheimer’s disease. Alzheimer’s and Dementia vol. 18 2669–2686 Preprint at https://doi.org/10.1002/alz.12756 (2022).
- Tahami Monfared, A. A., Byrnes, M. J., White, L. A. & Zhang, Q. Alzheimer’s Disease: Epidemiology and Clinical Progression. Neurology and Therapy vol. 11 553–569 Preprint at https://doi.org/10.1007/s40120-022-00338-8 (2022).
- Seibert, M. et al. Efficacy and safety of pharmacotherapy for Alzheimer’s disease and for behavioural and psychological symptoms of dementia in older patients with moderate and severe functional impairments: a systematic review of controlled trials. Alzheimers Res Ther 13, (2021).
- Bai, B. et al. Proteomic landscape of Alzheimer’s Disease: novel insights into pathogenesis and biomarker discovery. Molecular Neurodegeneration vol. 16 Preprint at https://doi.org/10.1186/s13024-021-00474-z (2021).
- Sperling, L. E., Klaczinski, J., Schütz, C., Rudolph, L. & Layer, P. G. Mouse acetylcholinesterase enhances neurite outgrowth of rat R28 cells through interaction with laminin-1. PLoS One 7, (2012).
- van Dyck, C. H. et al. Lecanemab in Early Alzheimer’s Disease. New England Journal of Medicine 388, 9–21 (2023).
- Vaz, M., Silva, V., Monteiro, C. & Silvestre, S. Role of Aducanumab in the Treatment of Alzheimer’s Disease: Challenges and Opportunities. Clinical Interventions in Aging vol. 17 797–810 Preprint at https://doi.org/10.2147/CIA.S325026 (2022).
- Hampel, H. et al. The Amyloid-β Pathway in Alzheimer’s Disease. Molecular Psychiatry vol. 26 5481–5503 Preprint at https://doi.org/10.1038/s41380-021-01249-0 (2021).
- Husain, M. A., Laurent, B. & Plourde, M. APOE and Alzheimer’s Disease: From Lipid Transport to Physiopathology and Therapeutics. Frontiers in Neuroscience vol. 15 Preprint at https://doi.org/10.3389/fnins.2021.630502 (2021).
- Milà-Alomà, M. et al. Plasma p-tau231 and p-tau217 as state markers of amyloid-β pathology in preclinical Alzheimer’s disease. Nat Med 28, 1797–1801 (2022).
- Li, Q. X. et al. GLP-1 and Underlying Beneficial Actions in Alzheimer’s Disease, Hypertension, and NASH. Frontiers in Endocrinology vol. 12 Preprint at https://doi.org/10.3389/fendo.2021.721198 (2021).
- Amadoro, G. et al. Nerve Growth Factor-Based Therapy in Alzheimer’s Disease and Age-Related Macular Degeneration. Frontiers in Neuroscience vol. 15 Preprint at https://doi.org/10.3389/fnins.2021.735928 (2021).
- Rohn, T. T., Rissman, R. A., Davis, M. C., Kim, Y. E., Cotman, C. W., & Head, E. Immunohistochemical analysis of caspase expression in the brains of individuals with obesity or overweight. Neurobiology of disease 11(2), 341-354 (2002).
- Foster, E. M., Dangla-Valls, A., Lovestone, S., Ribe, E. M. & Buckley, N. J. Clusterin in Alzheimer’s disease: Mechanisms, genetics, and lessons from other pathologies. Frontiers in Neuroscience vol. 13 Preprint at https://doi.org/10.3389/fnins.2019.00164 (2019).
- Bennet, A. M. et al. Genetic association of sequence variants near AGER/NOTCH4 and dementia. Journal of Alzheimer’s Disease 24, 475–484 (2011).
- Noura, M. et al. Pivotal role of DPYSL2A in KLF4-mediated monocytic differentiation of acute myeloid leukemia cells. Sci Rep 10, (2020).
- Henjum, K. et al. Cerebrospinal fluid catecholamines in Alzheimer’s disease patients with and without biological disease. Transl Psychiatry 12, (2022).
- Lauretti, E., Dincer, O. & Praticò, D. Glycogen synthase kinase-3 signaling in Alzheimer’s disease. Biochimica et Biophysica Acta - Molecular Cell Research vol. 1867 Preprint at https://doi.org/10.1016/j.bbamcr.2020.118664 (2020).
- Gao, J. et al. Icariside II, a phosphodiesterase 5 inhibitor, attenuates cerebral ischaemia/reperfusion injury by inhibiting glycogen synthase kinase-3β-mediated activation of autophagy. Br J Pharmacol 177, 1434–1452 (2020).
- Hampel, H. et al. The β-Secretase BACE1 in Alzheimer’s Disease. Biological Psychiatry vol. 89 745–756 Preprint at https://doi.org/10.1016/j.biopsych.2020.02.001 (2021).
- Du, H., Meng, X., Yao, Y., & Xu, J. The mechanism and efficacy of GLP-1 receptor agonists in the treatment of Alzheimer’s disease. Frontiers in Endocrinology 13, 1033479 (2022).
- Endicott, S. J., Ziemba, Z. J., Beckmann, L. J., Boynton, D. N. & Miller, R. A. Inhibition of class I PI3K enhances chaperone-mediated autophagy. J Cell Biol 219, (2020).
- Zhang, J. et al. Graphene oxide improves postoperative cognitive dysfunction by maximally alleviating amyloid beta burden in mice. Theranostics 10, 11908–11920 (2020).
- Vojdani, A. & Vojdani, E. Amyloid-Beta 1-42 Cross-Reactive Antibody Prevalent in Human Sera May Contribute to Intraneuronal Deposition of A-Beta-P-42. Int J Alzheimers Dis 2018, (2018).