What are the health problems that your research focuses on?
One of the big issues currently faced throughout the world is the rise in noncommunicable diseases. This suite of diseases is linked to the way that we choose to live our lives, and one of the biggest factors in that is what we chose to eat. An unhealthy diet has been highlighted as a major problem in the majority of these diseases, such as obesity, cancer, cardiovascular diseases, and type 2 diabetes.
It is estimated that there will be 629 million adults with diabetes by the year 2045. The vast majority of diabetes is type 2 diabetes. This is a lifestyle problem; it increases when obesity increases in the background population. And a big concern is the rise in diabetes in low-to-middle income countries. There is an enormous problem in the world about the way that we choose to live our lives and the way that we select the food that we eat.
These diseases show no sign of slowing down. No country in the world has been able to reverse the rise in obesity. There has been a massive increase in lifestyle diseases over very few years. So, this is not a genetic problem; this is an environmental problem. It is due to the vast change in our lifestyles and the increase in energy-dense foods that we choose to eat. Now 30% of the global population are overweight and obese, and this is expected to rise.
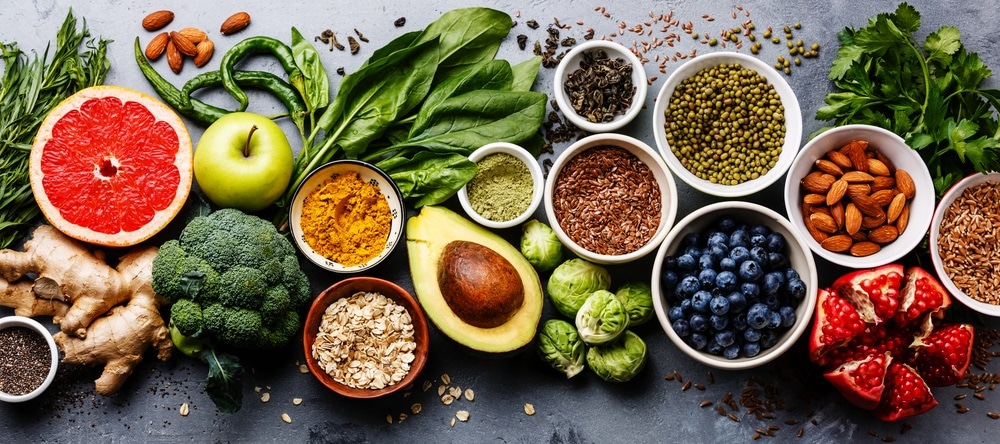
Image Credit:Shutterstock/Natalia Lisovskaya
What are the barriers to dealing with these issues?
The human race has made many big discoveries, but the one thing that we have not cracked is understanding what people eat in their home environment. At the moment, it's a total mystery about what people choose to eat on a day-to-day basis in their home environment. This is fundamentally important because, unless we can get this understanding, it is very difficult to link disease rates to diet. It is also very difficult to understand the success of a policy, because you don't know what people are eating on a day-to-day basis.
One of the big challenges in nutrition is to fix this problem. We want to answer the question: "What are we really eating?" Our current tools that we use to try and answer this are archaic. They are tools that have been around for over a century now. People record what they have to eat, and that is then processed using software. The problem with this is that there are errors along the way. One of the biggest is around what people choose to let you know that they eat. There is somewhere between a 33% to an 80% error rate in dietary reporting in some of the big data sets that we have, which governments use to make decisions on public health policy.
What we have done over the last six or seven years is to look at whether we can use 1H-NMR metabolite profiling in urine to give us insight into the diet.
How did you develop this 1H-NMR metabolic profiling technique?
We began by running a clinical trial where we put people under surveillance to make sure they had 100% adherence to our diet. We had 19 healthy participants follow, in a random order, four different diets with different levels of adherence to the WHO dietary guidelines. In a summary, we had a diet with 25% adherence to WHO guidelines and one with 100% adherence, plus two intermediate ones, with 50% and 75% adherence, respectively.
All of the participants followed all of the diets, but for the duration of each diet, participants were not allowed to leave the clinical unit, and all of the food and drink that we provided to them was controlled. All of the participants received the same three main meals and two snacks each day. Although, of course, these meals were different in each of the different diets.
Participants were asked to collect urine samples at the beginning and the end of each of the diet. We analyze the urine samples using nuclear magnetic resonance, and it takes five minutes to generate a metabolic profile or metabolic fingerprint. The metabolic profile can detect thousands of chemical compounds. The spectra contains many different peaks, and some of them are related to the intake of specific foods. For example, if a person consumes grapes, you will see tartaric acid in the urine. If they consume a piece of red meat, you will see O-acetylcarnitine.
We collected all of the urine samples from the participants after following each of the diets. We then took all of the metabolic profiles and put them in our model using PCA (principle component analysis).
What were your initial findings?
We found that even though the model does not know is who is who, or who has been eating which diet, our samples ranked according to the healthiness of the diet.
Essentially, what we found is that the participant's metabolic profile was systematically modulated when there is a change of diet, from a 25% to 100% healthy diet and vice versa. Another important factor in metabolic profiling is that it allows us to rapidly track changes in people's dietary habits. We were able to see changes in the metabolic profiles from day 1 to day 2 to day 3.
We plotted out results from people on the most and the least healthy diets with each sample represented by a dot. This showed a clear separation between the urine samples after people followed the healthy diet and after people followed the unhealthy diet.
We then wanted to see if we could use this model to predict the metabolic profiles of people who were following the intermediate diet. This was indeed possible, with the intermediate diets clustering between the two extreme diets.
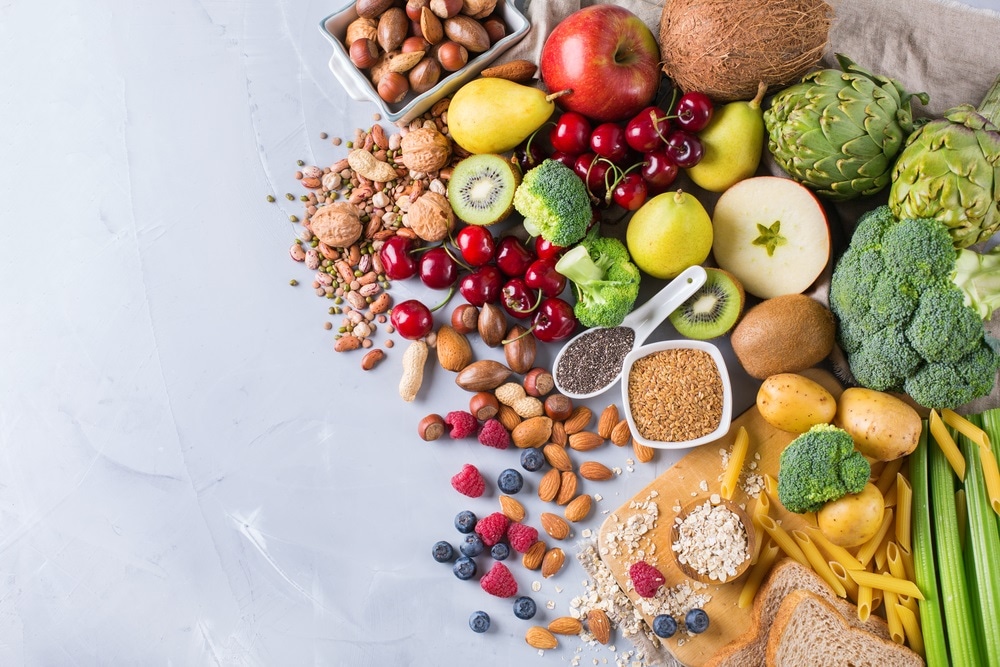
Image Credit:Shutterstock/ Antonina Vlasova
How did you validate the model outside the clinical-trial setting?
We took a group of free-living individuals that were classed as healthy eaters by a dietician. Dieticians look at what people say they eat, and they score them using the DASH (Dietary Approaches to Stop Hypertension) score. We plotted these DASH scores against our scores derived from metabolic profiling. We found that although the scores correlated for many of the participants, there was a significant proportion whose metabolic profiling indicated an “unhealthy” diet, despite a good DASH score.
Another advantage of our approach is that we can go into the metabolic profile and see why the DASH score is not matching our score. For example, one subject reported consuming a lot of orange juice and oranges, but we never saw proline betaine, a marker of citrus fruit consumption, in the urine. So, this was a case where someone has misreported or under-reported their dietary habits.
What components are you specifically looking for in the metabolic profile?
We are not necessarily looking for any specific compound in its own right. What we are doing is taking the whole pattern, the metabolic fingerprint of the urine. From this, we match it to a database of very carefully controlled dietary intervention studies.
That said, there are certain compounds in the urine that we do look at specifically because we know they are of interest. Some of these will include things like energy metabolites, involved in the Krebs cycle. Another group of compounds we are interested in is the gut microbial metabolites, because they relate specifically to the bacterial harvesting of calories. This includes metabolites like para-cresol sulfate, para-cresol glucuronide, phenylacetylglutamine, hippurate, as well as other metabolites deriving directly from foods themselves. For example, if you take proline betaine, it is reporting specifically on citrus fruit. So, we will give a readout of specific chemicals, but the whole premise of the test is around the global, holistic pattern in urine that we are using to make predictions.
What are the main advantages of the test?
From the clinic’s perspective, this test provides dieticians the opportunity of knowing what the person has been eating without having to ask them. We know that normally this has a huge error margin because they are misreporting or under-reporting. We believe we can empower dieticians to be able to personalize the diet, knowing what the person has been eating, but also, they will know which diet matches better the metabolism of the person. So, we can see how they respond to the diet.
From a scientific perspective, this approach allows researchers a different way of measuring what people have been eating, and not just relying on food questionnaires or food diary.
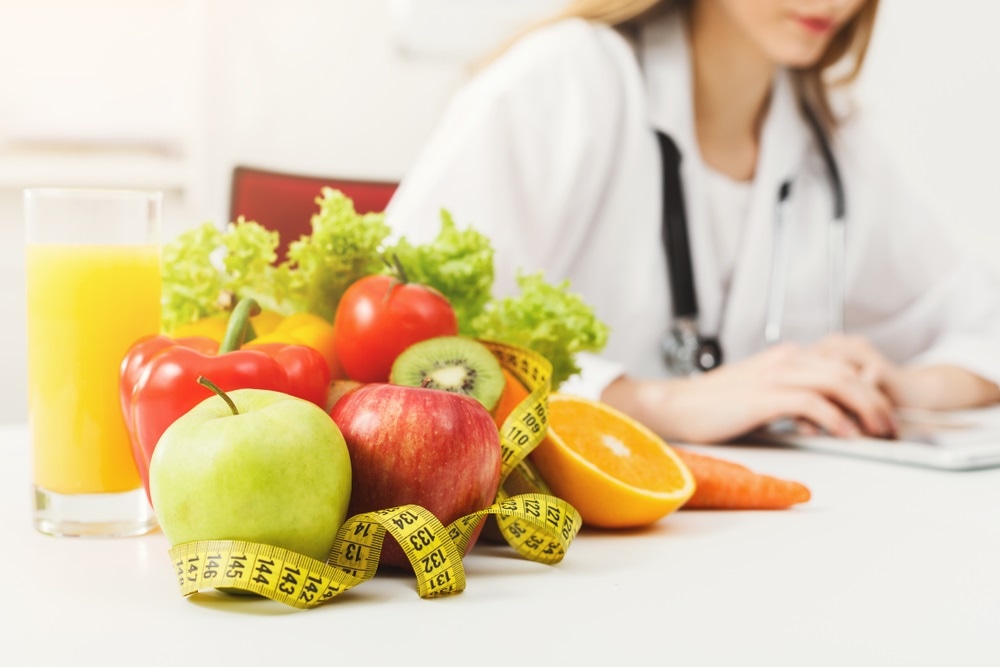
Image Credit:Shutterstock/Prostock-studio
How could the method be used to track people's dietary habits in real life?
We did another clinical trial, in which 28 volunteers were asked to collect a weekly urine sample over a period of six months. Once again, we ran the samples using nuclear magnetic resonance, we generated a metabolic profile, and we applied our mathematical model to be able to assess the adherence of each of the participants over time to the WHO guidelines.
For each participant, we generated a different plot so we could monitor their dietary changes over time. In the x-axis, we plotted the day when the urine sample was collected, and on the y-axis, we plotted the percentage of adherence to WHO guidelines for each sample. What we want to see, ideally, is a line at around 100% of adherence to WHO guidelines, but, in reality, it does not always happen. We saw that some people were starting with a very healthy diet and then losing adherence over time.
Finally, we applied our model to look at people's dietary habits in their home environment. We picked a group of obese patients who were due to undergo bariatric surgery. This time we plotted the percentage of adherence to WHO guidelines against the DASH score. We found that, in many cases, the DASH score was not in agreement with our adherence score.
There were some people that, according to our model, had better dietary habits. However, less than 50% of the subjects showed good adherence to healthy dietary habits.
How could this information tailor diets to individuals?
Some people think that if everybody follows the same diet, everybody will lose approximately the same amount of weight and the outcomes are to do with compliance. If you ask other researchers, they will say that is not the case and the diet should be tailored to your individual metabolism.
We have researched this over 20 years now and have done numerous studies where we fed people with single- and mixed-food diets. For example, if we take a healthy cohort who received no grapes in their diet when we analyze the urine sample, everybody starts with zero tartrates, our biomarker for grape consumption.
We then had people eat the same number of grapes per day, and what we saw is that everybody excretes a different amount of this particular biomarker. Some people excrete more than double the amount that other people do. So, what we have found is that although people receive exactly the same amount of the same food, there are differences in how those people metabolize and/or excrete the food. You can expand this concept through different foods. We have observed the same effect with different compounds related to red meat, fruits and vegetables, to fish, and broccoli, for example. For every single food type, we see a spread in response. So, people do metabolize different foods differently.
As an example, we can look at a particular biomarker, proline betaine, which is a biomarker for citrus fruit. It is a very good biomarker for us because it comes in an uncrowded region of the 1H-NMR spectrum, so we can easily detect it. And it is not metabolized in any major way to other chemicals; most of what you consume is excreted as proline betaine.
We fed some volunteers the same amount of orange juice, and then we profiled their urine at two, four, six hours, and so forth, up to 24 hours after drinking the orange juice. What we found is that, for proline betaine, most people excrete the majority within the first two hours, but you can still detect it up to 24 hours later. One thing is for certain is we can look at acute dietary responses. When we considered these acute dietary responses and apply them in a free-living population and look at dietary records, we were able to predict the people who were drinking orange juice.
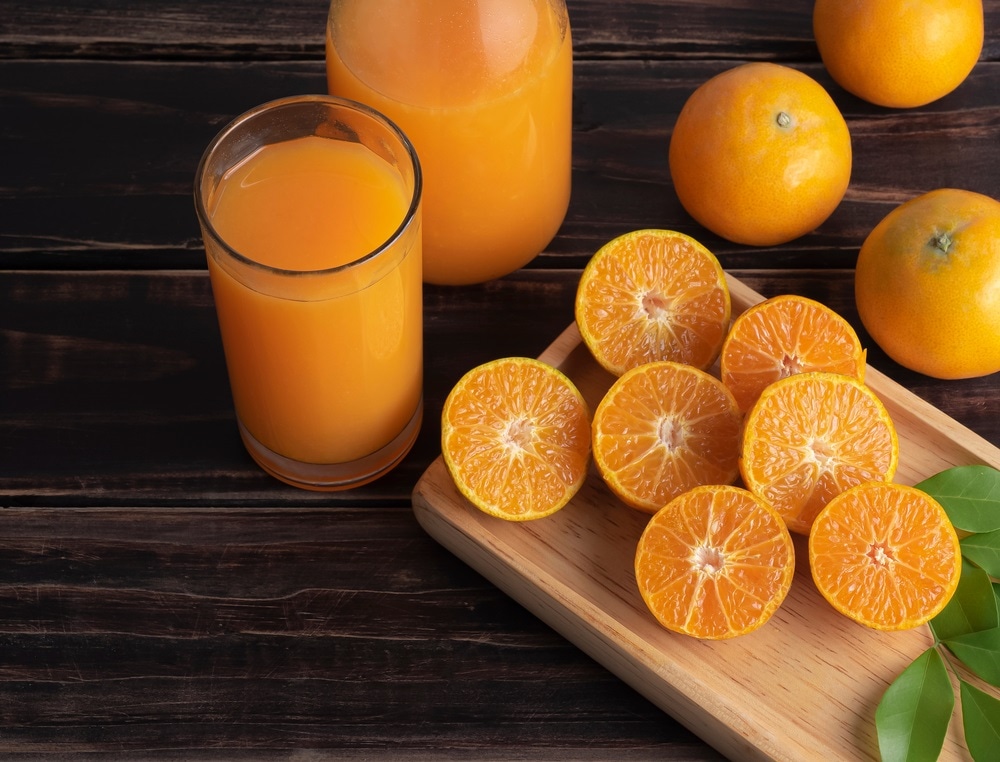
Image Credit:Shutterstock/ AtSkwongPhoto
Does this method allow you to look at the diet in a longer-term sense?
We have taken the data from our in-house study where we profiled the 19 people variety carefully. We identified the metabolites that differed between the good diet and the bad diet, and for each of the metabolites that changed across those two diets, we built them into a metabolic network and looked to see how these metabolites were connected across different people. We visualize these on a metabolic reaction network map.
This allows us to see, after following a particular diet, how a person's metabolism lights up across multiple pathways. If a person is not responding, that indicates they are not as metabolically active following this particular diet.
What determines whether someone becomes more metabolically active following a particular diet?
It differs between individuals, but at least some of those differences are down to the gut microbiome. A lot of what we eat is processed by our gut bacteria into chemicals, and these are excreted. So, some of the differences we see in the urine profiles are to do with the fact that each of us has about 1.5-2 kilograms of gut bacteria, and we are all unique. We have different bacteria. This makes us all slightly different, metabolically. The gut bacteria contribute to individual differences in our profile.
Now, if we are to think of a person throughout their lifespan, ideally, we want to maintain health for as long as possible, and the diet is a big part of that. For each population and each individual, it is key to be able to work out, what is the best diet for you?
About Dr. Isabel Garcia-Perez
Dr. Garcia-Perez is a Lecturer in Precision and Systems Medicine within the Division of Integrative Systems Medicine and Digestive Diseases. With her growing research, her aim is to exploit a new research niche that brings analytical chemistry to health research. Her dedication to the work that she does really sits her at the forefront as a role for Women in Science.
About Prof. Gary Frost
Prof. Frost is currently head of the Section for Nutrition Research and lead the Imperial Nutrition and Food Network. He is qualified as a dietitian in 1982 and have always maintained a clinical input throughout his career. He was appointed to Professor of Nutrition and Dietetics at Imperial College Jan 2008. Prior to this, for 18 years Gary had work at Hammersmith Hospital. Over his time at Hammersmith he gained his PhD in Nutrition and was appointed Honorary Reader in Nutrition at Imperial College, then joined the University of Surrey as Professor of Nutrition and Dietetics in 2005.
Prof. Elaine Holmes
Prof. Holmes is the Head of the Division of Computational and Systems Medicine and a Professor of Chemical Biology in the Department of Surgery and Cancer at Imperial College, in London, UK. She has over 20 years experience in metabonomic technology and its applications. Her focus is on the discovery and development of metabolic biomarkers of disease in personalised healthcare and population studies with significant contributions to cardiovascular, neuroscience and infectious disease research.