In a recent study posted to the bioRxiv* preprint server, the team of researchers explored the protein-protein interaction between the severe acute respiratory syndrome coronavirus 2 (SARS-CoV-2) non-structural protein 1 (Nsp1) and host 40S and 43S ribosomal subunits.
Study: SARS-CoV-2 Nsp1 N-terminal and linker regions as a platform for host translational shutoff. Image Credit: ATS / Shutterstock

*Important notice: bioRxiv publishes preliminary scientific reports that are not peer-reviewed and, therefore, should not be regarded as conclusive, guide clinical practice/health-related behavior, or treated as established information.
SARS-CoV-2, the causative organism for the ongoing coronavirus disease 2019 (COVID-19) pandemic, is a positive-sense single-stranded ribonucleic acid (RNA) virus similar to the other coronaviruses (CoV) identified. SARS-CoV-2 belongs to the beta genus of CoVs, which also comprises the Middle East respiratory syndrome (MERS-CoV) and SARS-CoV-1. COVID-19 is a highly pathogenic respiratory disease with mild to severe forms.
The inhibition of host protein synthesis is the characteristic feature of CoVs infection resulting in selective translation of some host proteins and the viral factors by the ribosomes. During the initial stages of COVID-19, the SARS-CoV-2 Nsp1 inhibits the host's innate immune responses by inserting its C-terminal helices into the messenger RNA (mRNA) entry site of the ribosome, thereby stimulating mRNA degradation. However, the exact mechanism through which the Nsp1 attains host translational shutoff and permits viral protein synthesis is not yet understood.
About the study
In the present study, the scientists characterized the whole set of molecular interactions of full-length Nsp1 and its topology on the 40S and 43S ribosomal subunits by crosslinking mass spectrometry (MS) to examine the role of the CoVs N-terminal domain (NTD) and linker regions in host translational shutoff.
The researchers developed an integrative model by incorporating the existing structural knowledge with the data regarding spatial limitations of Nsp1 derived from the crosslinking MS. The team further characterized the Nsp1-bound proteome by ultracentrifugation and affinity purification MS (AP-MS).
Findings
The results indicate that the NTD and linker regions of the SARS-CoV-2 interact with 40S ribosomal proteins present in the mRNA entry channel of the host. Further, a new interaction was identified between the NTD and linker regions and the G subunit in the RNA-recognition motif (RRM) domain of the eukaryotic initiation factors 3 (eIF3) complex.
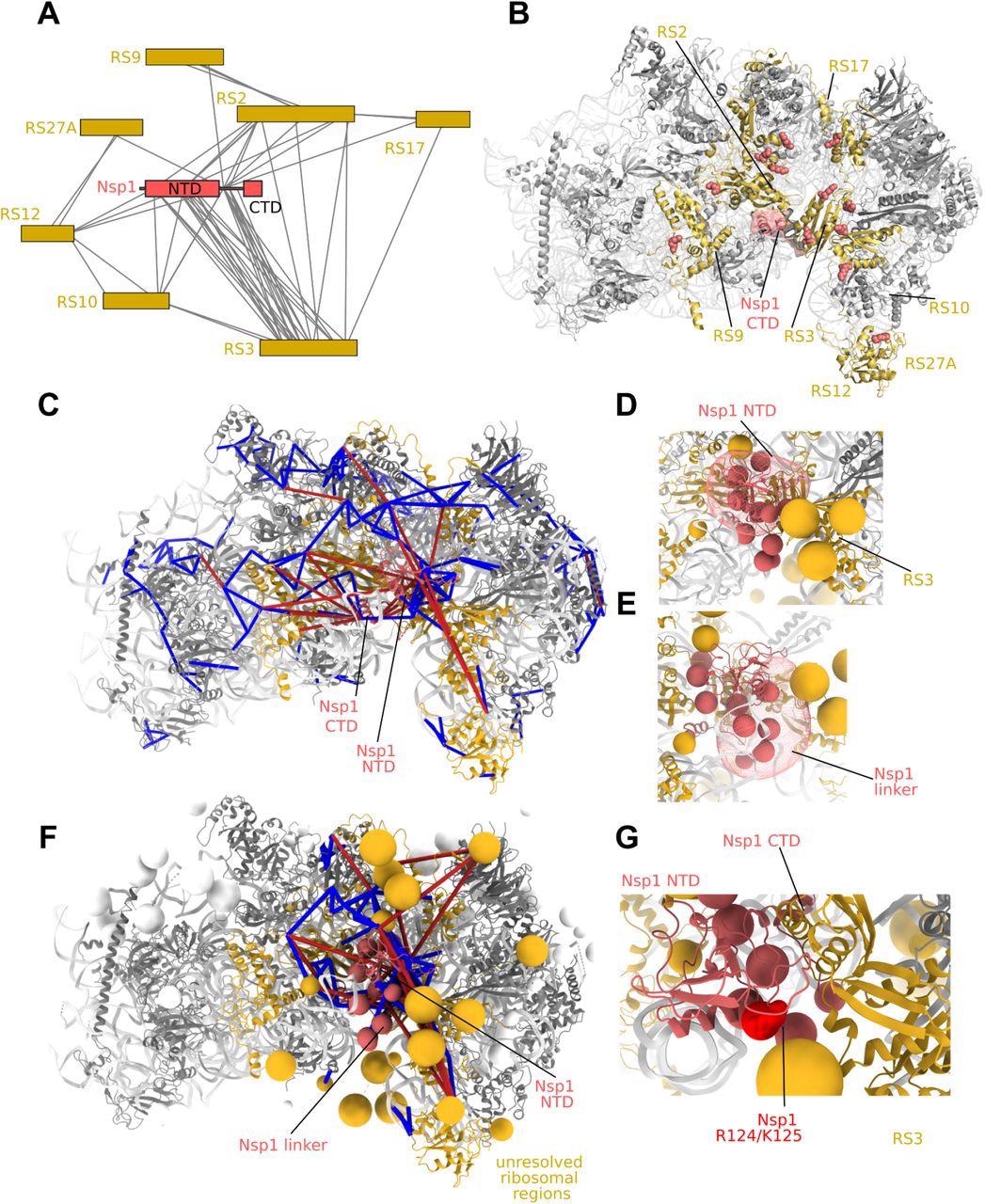
Protein-protein interactions of the Nsp1 NTD. A) Interactors of Nsp1 by crosslinking-MS. The Nsp1 NTD and linker regions are observed in a large number of interactions with ribosomal S3 (uS3). B) 40S subunit (pdb 6zlw) proteins interacting with Nsp1 highlighted in gold. Residues showing crosslinks to Nsp1 are shown as spheres. C) Integrative modeling of Nsp1 NTD onto the 40S ribosome. The solution represents the centroid of the most populated cluster of models, with the Nsp1 NTD interacting with RS3. Crosslinks are mapped onto the complex. Satisfied crosslinks (<30Å) in blue, violated crosslinks are in red. The crosslinks show secondary populations where the Nsp1 NTD samples the other side of the cavity facing the mRNA entry site. D) Localization probability density of the Nsp1 NTD in the main cluster of models. Beads comprising the coarse-grained regions not present in the structure (Nsp1 linker, flexible ribosomal protein regions) are shown. E) Localization probability density for the Nsp1 linker region in the main cluster. The linker largely localizes at the bottom of RS3. F) Integrative modeling result with all coarse-grained regions displayed. All Nsp1 crosslinks are shown, including those to coarse-grained regions. G) In the main cluster of models, K125 is observed in close proximity to RS3.
The localization probability of the SARS-CoV Nsp1 linker region demonstrated that the linker region has a selective affinity for localization on the right side of the ribosomal entry site cavity. The deletions in the K141-S142 linker residues of SARS-CoV Nsp1 diminish the translation shutoff function in CoV Nsp1 and may result in a drop in ribosome binding capacity.
An integrative model was derived with full-length Nsp1 on the 40S subunit targeting the dynamic interface between the Nsp1, eIF3, and ribosome complex via the crosslink-derived distance limitations.
The crosslinking-MS network indicated that eIF3G crosslinked to ribosomal subunits G1, A1, and A2 of the eIF4F complex and showed a direct crosslink between eIF4B and Nsp1.
Additionally, it was observed that Nsp1 predominantly binds with the 40S and 43S preinitiation complexes, probably due to the RNA-mediated or direct interaction between Nsp1 and eIF3G indicated by the proteomic analysis. The crosslinks provide clarifications for the existing structural models of Nsp1-bound ribosomes and initiation complexes by providing alternative conformations for the eIF3B-eIF3I module uniquely using the eIF3G RRM.
Since Nsp1 interaction occurs with regions containing the NXF1 RRM domain indicated by the AP-MS protocol, it further supported the ability of Nsp1 to interact with RRM domains. The fluorescence-based measurements demonstrated that Nsp1 and eIF3J exhibited a competition for binding with the 40S ribosomal subunit.
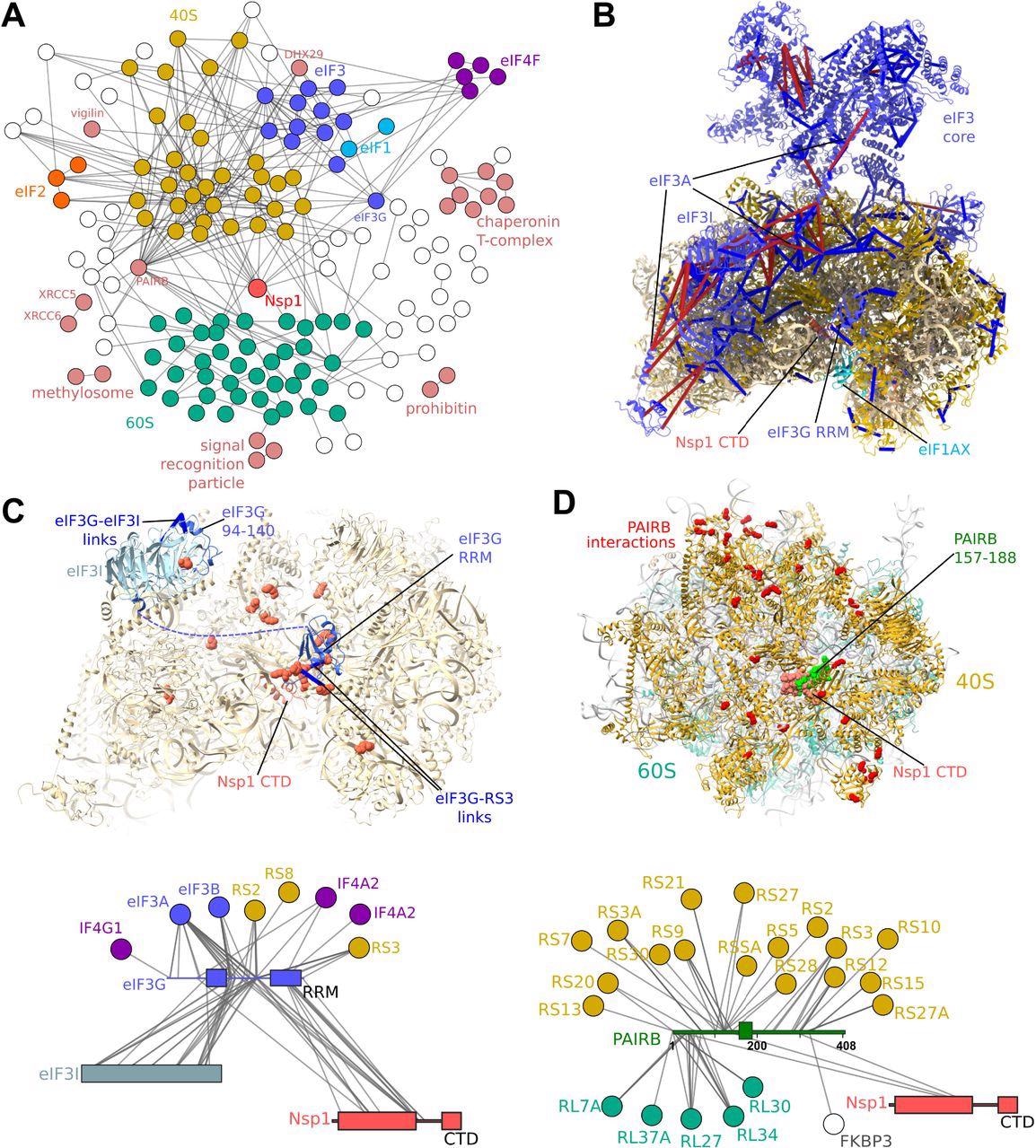
Protein-protein interaction map of the Nsp1-bound proteome. A) Crosslinking-MS detects 515 protein-protein interactions (PPIs) at a 6% PPI-level FDR. Each edge represents one or more crosslinks. Nsp1 primarily interacts with the 40S and eIF3 complexes. Additional interactions between the ribosome and regulatory or initiation factors are detected. B) Crosslinks mapped onto the structure of the Nsp1-bound 43S initiation complex (pdb 6zp4) (10). Satisfied crosslinks (<30Å) in blue, violated crosslinks are in red. The crosslinks are largely consistent with the structure, with violations highlighting the mobility of the head region of eIF3, and of the eIF3I subunit. Crosslinks indicate a possible alternative register for the long helix of eIF3A, which was poorly resolved in the structure. C) Crosslink-guided assignment of the unidentified RRM domain in the complex, and modeling of the eIF3I-binding region of eIF3G by homology and crosslinking-MS data. 40S and eIF3G-RRM residues cross-linked to Nsp1 NTD and linker regions are shown as red spheres. D) PAIRB binds Nsp1-stalled ribosomes and 40S subunits View of the face of the 40S subunit with residues cross-linked to PAIRB shown as red spheres. Crosslinks show the large, disordered protein wraps around the 40S subunit, with its plug region displaced by the Nsp1 helices. Crosslinks mapped onto PAIRB-bound 80S ribosome (pdb 6z6n) (46). Crosslinks also show interactions between Nsp1 NTD and PAIRB.
Conclusions
The study findings demonstrate the high conservation of the Nsp1 NTD and linker in CoVs. Further, it indicates the involvement of CoVs NTD and linker in a vast number of protein-protein interactions.
The present model summarizes the previous cryogenic electron microscopy (cryo-EM) studies and the reasons for the absence of ribosomes in the Nsp1-bound proteome in prior investigations, possibly due to the high background of the ribosome or the use of C-terminal tags. Thus, it provides a framework to understand the impacts of previously reported deletions in protein conformational dynamics.
The authors assume that since translation initiation on a viral transcript may ensue through an eIF3G-independent mechanism, it partially justifies the selectivity of CoVs Nsp1 for host-translating ribosomes. Further, the Nsp1-eIF3G interaction keeps the former protein off the ribosome.
Overall, the study reveals that a mechanism via Nsp1 is mainly recruited to the established initiation complexes resulting in selective inhibition of host-translating ribosomes and the subsequent degradation of mRNA.

*Important notice: bioRxiv publishes preliminary scientific reports that are not peer-reviewed and, therefore, should not be regarded as conclusive, guide clinical practice/health-related behavior, or treated as established information.