Sponsored Content by MaxCyte, Inc.Reviewed by Maria OsipovaOct 11 2023
In this interview, NewsMedical talks to James Brady about cell-based therapy and how the field of genetic engineering transformed.
How have recent breakthroughs in genetic engineering propelled cell-based therapeutics to the forefront of medical advancements?
Early cell-based therapies that relied on non-engineered cells often showed low therapeutic efficacy due to rapid clearance of transfused cells or ineffective interactions between effector and target cells.
Many of those therapeutic shortcomings have been addressed through recent advances in non-viral gene delivery technologies and precision gene editing tools, such as CRISPR, base editing and prime editing.
Now, we can engineer immune cells to target tumors by introducing genes that express antigen-specific receptors, and we can increase therapeutic potency by knocking out genes that encode checkpoint inhibitors or non-self HLA molecules. We can also correct disease-causing mutations or augment the cellular repair capabilities of stem cells for regenerative medicine applications.
What is electroporation, and how has this technique revolutionized genetic engineering?
Electroporation is a technique for creating temporary perturbations to the cell membrane through the brief application of electrical pulses. There are multiple applications for electroporation, but in the context of cell therapy, electroporation is primarily used for introducing genetic material and other molecules into cells.
Electroporation is very powerful because it is compatible with a wide range of cell types and loading agents, it enables complex manipulations of cellular function, and it offers greater scalability and consistency than other transfection methods. Moreover, with the appropriate instrumentation, the technology provides regulatory compliance, and it can be used in the clinic with minimal impact on the viability of patients’ cells.
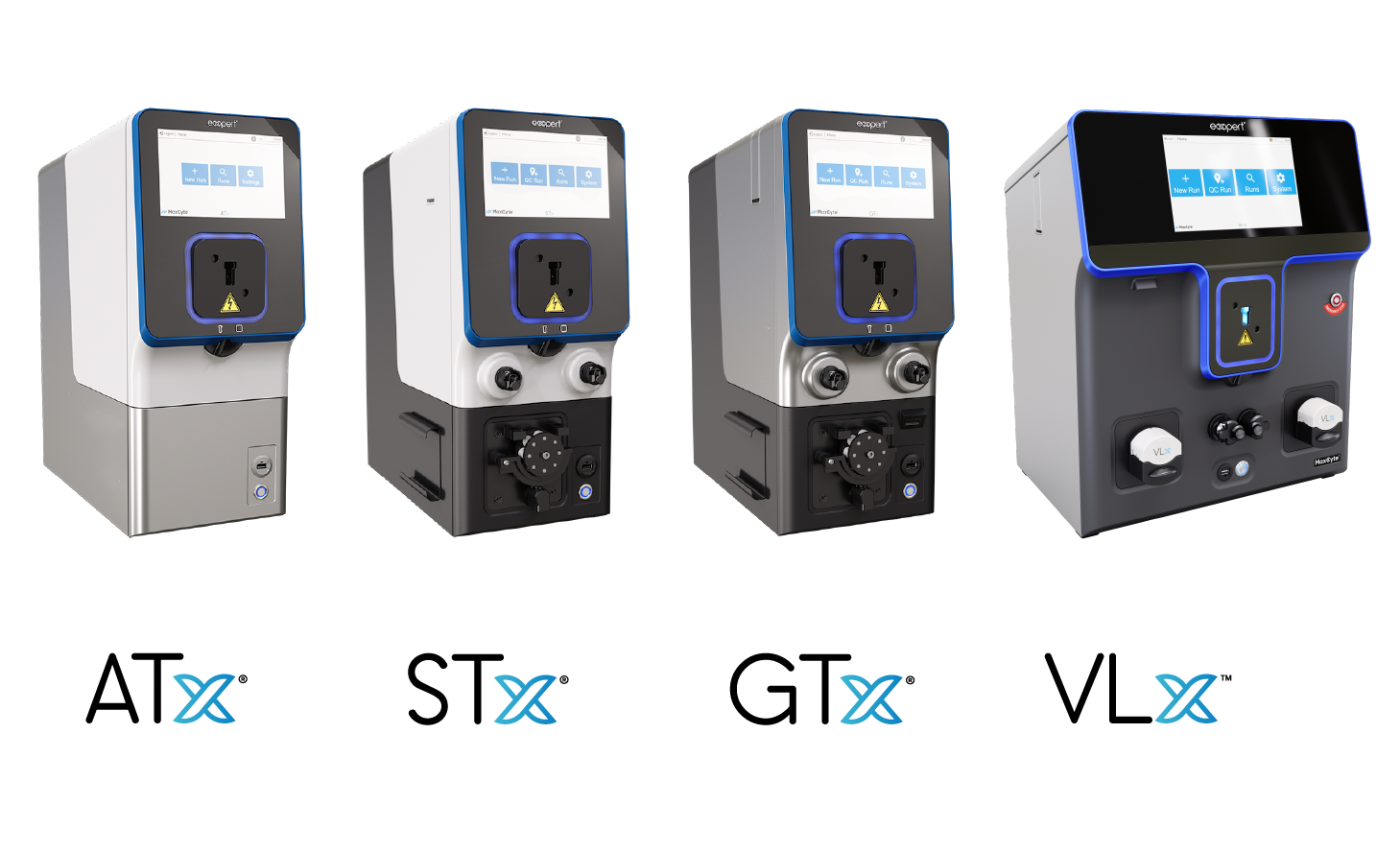
Image Credit: MaxCyte, Inc.
Could you shed light on the role of electroporation in cell engineering for therapeutic purposes?
Electroporation can introduce genetic sequences composed of nucleic acids into the cell to produce proteins that augment or improve cellular functions. Alternatively, molecules can be introduced to delete or alter endogenous genetic sequences using CRISPR and other gene editing technologies.
Some applications for gene editing include correcting mutations that cause diseases or preventing the expression of proteins that suppress the activity of immune cells.
What challenges in cell therapy development does MaxCyte® electroporation help researchers overcome?
First, MaxCyte’s electroporation technology provides an alternative to gene delivery with viral vectors, which are costly to manufacture and present challenges associated with random genomic integration and potential immune reactions to viral components.
Second, in contrast to other transfection technologies that are compatible with only a limited range of cells and loading agents, MaxCyte electroporation facilitates complex cellular engineering strategies based on introducing large molecules and combinations of molecules into a wide range of primary cell types, including blood cells and stem cells.
Finally, MaxCyte’s electroporation platform offers truly seamless process scalability. Results generated in a research lab using a few million cells can be translated to the clinic using billions of cells without performing complex re-optimization studies.
Can you explain how electroporation enables the efficient delivery of various genetic payloads into cells and why this versatility is important?
One major advantage of electroporation is that it allows users to control desired levels of transfection efficiency and cellular viability by changing the electroporation protocol and titrating loading agent concentrations.
Also, in contrast to reagent-based transfection methods, electroporation takes place in less than one second, so the timing of transfection can be controlled. This is important for applications where prolonged exposures to loading agents or transfection reagents may have undesired biological consequences.
In the context of cell engineering, why choose electroporation over other transfection techniques, such as viral vectors and lipid vesicles?
Electroporation allows for the simultaneous introduction of multiple types of molecules, including RNPs, large DNA molecules, messenger RNAs, and smaller oligonucleotides or siRNAs. This versatility is generally not provided by other transfection technologies, including lipid vesicles, which are typically formulated to accommodate only specific categories of loading agents.
As mentioned earlier, electroporation has several advantages over viral vectors, which are impacted by high manufacturing costs, lack of control over genomic integration, and potential immunogenicity.
What is flow electroporation, and how does it work?
Traditional electroporation is referred to as static electroporation because most electroporation instruments do not allow for the movement of cells to and from the electroporation chamber during the transfection process, limiting the number of cells that can be processed at one time.
In contrast, flow electroporation can transfect billions of cells during a single run based on a process that employs stepwise and gentle pull of smaller fractions into the electroporation chamber. All of the fractions are processed identically, and they are pooled into one consistent batch at the end of the operation.
The movement of cells is fully controlled by the instrument’s software and hardware. Automated cell movement provides much greater scalability and process standardization compared to static electroporation.
Also, with MaxCyte’s Flow Electroporation® technology, cells are drawn into the chamber via gentle negative pressure achieved by pumping air. Thus, the cells are never exposed to the shear stress of being compressed by peristaltic pumps, and high cell viability is maintained.
How does Flow Electroporation streamline cell therapy production, and what benefits does this offer to researchers and patients?
MaxCyte’s Flow Electroporation technology can transfect billions of cells in a single batch, enabling the manufacturing of both autologous and allogeneic cellular therapies. Additionally, MaxCyte’s electroporation instruments can be integrated into a fully closed, GMP-compliant cell engineering workflow.
Both of these advantages, scalability, and closed workflow integration, overcome the shortcomings of conventional static electroporation and reagent-based transfection methods, which typically involve multiple manual manipulation steps that introduce variability and other sources of error.
Can you elaborate on using electroporation for CAR T cell development and its impact on patient treatment?
CAR T therapies are becoming increasingly sophisticated. Aside from the introduction of the CAR expression cassette, CAR T manufacturing often involves additional cellular engineering steps, including the knockout of genes that encode checkpoint inhibitors, endogenous receptors and target antigens.
While the CAR gene is often delivered virally, gene knockout is typically accomplished via electroporation. Moreover, there is a growing trend to introduce CAR genes by electroporation, either by employing transposons in place of viral vectors or by integrating the CAR via CRISPR-mediated homologous recombination.
Thus, electroporation provides multiple benefits for patients by enabling therapeutic strategies that yield greater efficacy through higher potency, consistent scalability, and increased persistence of transfused cells.
The German Cancer Research Center (DKFZ) has recently used MaxCyte electroporation in an automated protocol for producing recombinant T-cells. Could you explain the advantages of using electroporation in this approach?
DKFZ developed a non-viral approach to CAR expression using non-integrating DNA vectors that are introduced into patients’ T cells via MaxCyte’s electroporation technology.
In addition to overcoming the limitations of viral gene delivery discussed earlier, MaxCyte’s GMP-compliant electroporation technology provided DKFZ with scalability, closed connectivity with upstream and downstream process steps, and improved control over process outcomes. Finally, the DKFZ group published data demonstrating that MaxCyte electroporation produced fewer changes to gene expression patterns in T cells compared to lentiviral CAR delivery.
Image Credit: MaxCyte, Inc.
In Carl June’s study that used MaxCyte electroporation to deliver CRISPR RNPs, what were the key findings and implications for improving T-cell therapies in clinical trials?
The paper from Dr. June’s group at the University of Pennsylvania described a first-in-human, phase 1 clinical trial that demonstrated the feasibility of multiplex CRISPR editing, enabled by MaxCyte electroporation, to improve the therapeutic efficacy of T cells that were also engineered to express a tumor-targeting T cell receptor.
By knocking out genes encoding the endogenous T cell receptor alpha and beta chains and the PD-1 checkpoint inhibitor, the cells showed greater in vitro cell-killing activity. Evaluation of several patients showed extended persistence of the engineered cells with no evidence of clinical toxicities.
How does MaxCyte accelerate innovative research into clinical applications?
A key advantage of MaxCyte’s approach to electroporation is that the same instrument can be used for small-scale, static electroporation with a few million cells and for clinical-scale Flow Electroporation with billions of cells.
This allows data and protocols to be seamlessly translated from the research lab into a GMP-compliant clinical process, eliminating the need for lengthy re-optimization studies.
Moreover, MaxCyte’s technology, which was originally developed for clinical use, has been validated in dozens of clinical trials for a wide range of therapeutic applications. Aside from providing best-in-class technology, MaxCyte gives customers and partners access to a highly skilled team of scientific, engineering, and regulatory experts who will guide users through all phases of therapeutic development.
About James Brady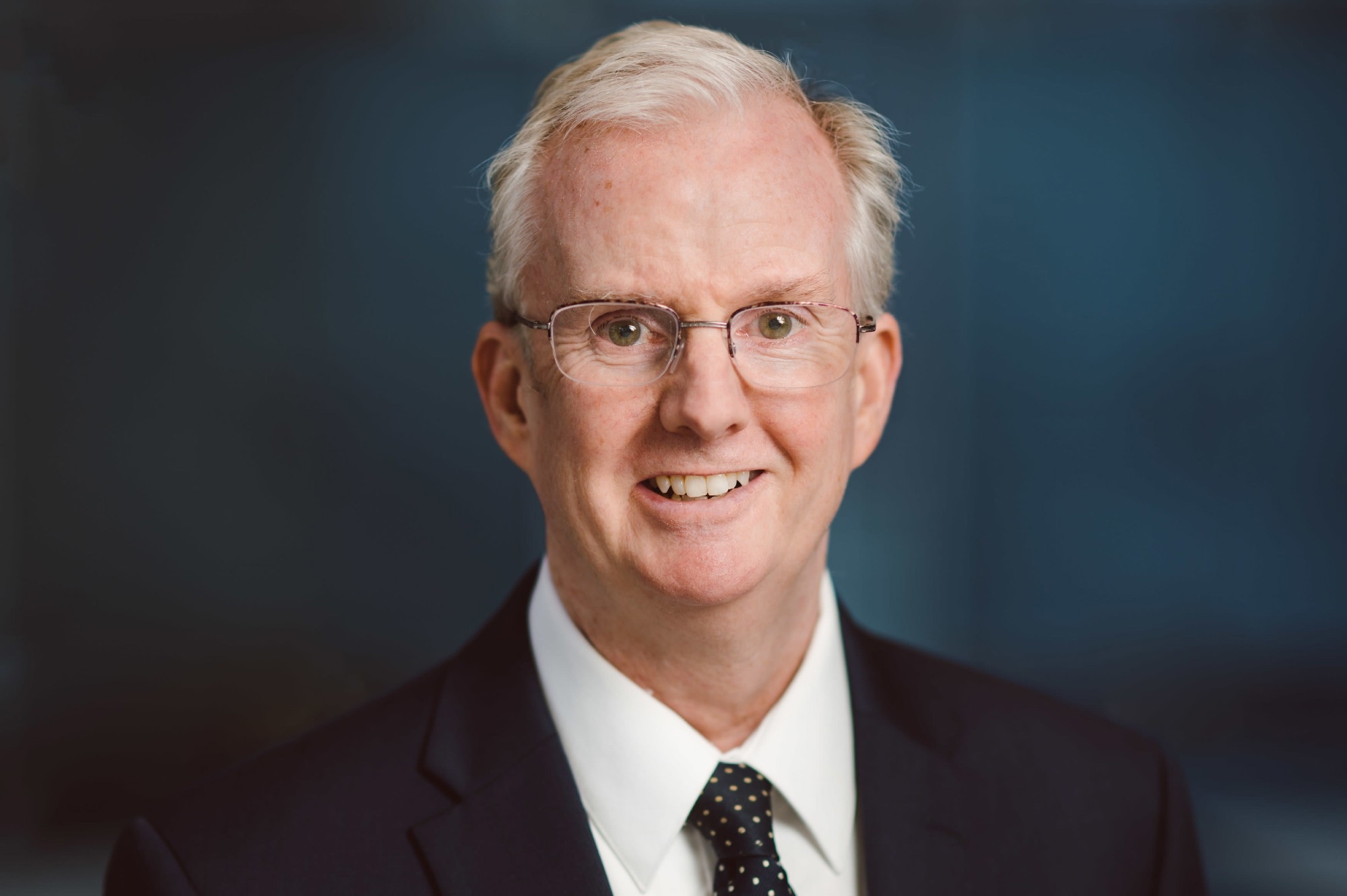
Dr. Brady is an experienced biotechnology industry professional with expertise in gene and cellular therapy, biologics, and drug discovery. Previously, he was a Senior Scientist at Genetic Therapy, a Novartis subsidiary, where he worked on lentiviral-based gene therapy treatments and a Group Leader at MetaMorphix, managing the company’s transgenic and genetic research programs.
Dr. Brady earned a Master of Business Administration in finance from The Johns Hopkins University. He completed his postdoctoral fellow at the National Eye Institute of the National Institutes of Health in Bethesda, Maryland after obtaining a PhD in genetics from Indiana University in Bloomington, and a Bachelor of Science in biology from the College of William and Mary in Virginia.
About MaxCyte, Inc.
MaxCyte is a leading commercial cell-engineering company focused on providing enabling platform technologies to advance innovative cell-based research as well as next-generation cell therapy discovery, development and commercialization. Over the past 20 years, we have developed and commercialized our proprietary Flow Electroporation® platform, which facilitates complex engineering of a wide variety of cells.
Our ExPERT™ platform, which is based on our Flow Electroporation technology, has been designed to support the rapidly expanding cell therapy market and can be utilized across the continuum of the high-growth cell therapy sector, from discovery and development through commercialization of next-generation, cell-based medicines. The ExPERT family of products includes: four instruments, the ATx™, STx™, GTx™, and VLx™; a portfolio of proprietary related processing assemblies or disposables; and software protocols, all supported by a robust worldwide intellectual property portfolio.