Sponsored Content by Pion IncReviewed by Maria OsipovaMay 5 2025
In this interview, Prof Na Li explores the mysteries of the particle drifting effect and its practical applications in real-world scenarios.
Could you explain why solubility is such a crucial factor in drug absorption?
Only solubilized drugs can be absorbed by the body. However, the poor solubility of many drugs—both currently on the market and those in development—has been a major challenge for the pharmaceutical industry.
As formulation scientists, we aim to develop strategies that enhance drug solubility to ensure effective absorption. Without these strategies, taking life-saving drugs would be no different from consuming a piece of brick—there would be no absorption and, consequently, no health benefit.
Is there a limit to how much the drug can dissolve in the solution?
Yes. While solubility enhancement strategies are powerful, they do not allow for infinite dissolution. Poorly soluble drugs have an upper concentration limit, which we call amorphous solubility. This is essentially the miscibility gap between the drug and water. Even if we dissolve more drugs into the solution, any amount beyond this amorphous solubility will precipitate out as a second phase, usually in the form of amorphous drug nanoparticles.
You discussed how amorphous nanoparticles form, but do they contribute to drug absorption?
Based on Dr. Sugano’s work, we already know that particle forms do contribute to absorption. The key reason is that the unstirred water layer represents a major barrier to the absorption of poorly soluble drugs.
Think of drug absorption like a hiking journey—to reach our destination (absorption), we must cross the unstirred water layer. Even if there is a large population of drug molecules in bulk solution, only a few can naturally diffuse through this barrier.
When small drug nanoparticles or bile micelles are present, they can act as vehicles, carrying large amounts of free drug across the unstirred water layer. These particles effectively drop off the drug molecules right at the intestinal wall, allowing faster and more efficient absorption. This was termed as the particle drifting effect.
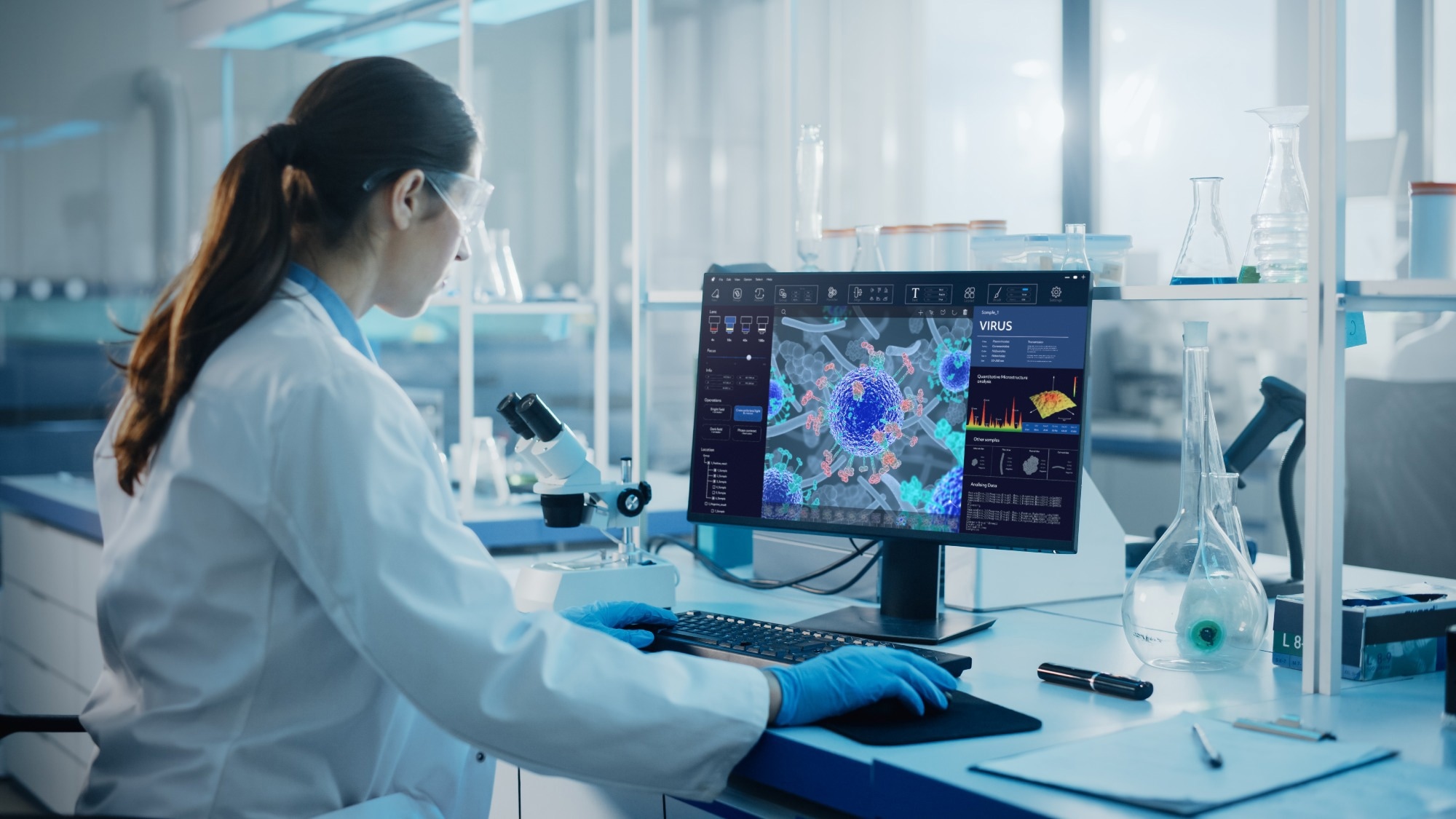
Image Credit: Gorodenkoff/Shutterstock.com
Can you explain how you measured the absorption advantages provided by these colloidal drug particles?
We used a biphasic diffusion setup to measure the flux and calculated effective unstirred water layer thickness (inversely proportional to flux). In this setup, we have an aqueous phase where we introduce the drug and the particles, and we measure the appearance of the drug in the organic phase over time. To quantify the particle drifting effect, we used differential equations to derive an expression for the thickness of the unstirred water layer.
We evaluated flux provided by amorphous drug nanoparticles and normalized the data against the flux of the free drug. We analyzed our data by plotting the effective permeability coefficient (which represents drug absorption in the organic phase) against the solubility of the drug composing the nanoparticles. This revealed a strong linear correlation. According to the Whitney-Noyes equation, the dissolution rate of a particle is proportional to the solubility of the drug that makes up the particle.
This suggests that the "particle drifting effect" is closely related to dissolution rate. Particles must dissolve first, release the drug at the membrane surface, and then the free drug can be absorbed. For highly soluble drugs like telaprevir, dissolution happens quickly, leading to a higher particle drifting effect. Conversely, for extremely insoluble drugs like anacetrapib, dissolution is much slower, resulting in only a modest permeability enhancement, even when particle size and concentration remain constant.
How does particle size affect the permeation behavior of different drugs?
We created particles of different sizes to examine the impact of particle size on two drugs, atazanavir and anacetrapib. For atazanavir, we saw no noticeable difference in permeation, regardless of particle size.
However, for anacetrapib, smaller particles led to significantly faster permeation compared to larger ones. This is expected, as smaller particles dissolve faster, while larger particles dissolve more slowly.
The difference we observed in these two drugs comes down to the mechanism of the particle drifting effect, which involves two sequential steps: dissolution and permeation. In the case of anacetrapib, which is highly insoluble, dissolution is very slow and becomes the rate-determining step of the overall process. By modifying the particle size, we effectively tune the dissolution rate, which in turn influences the overall reaction rate.
Atazanavir, which has higher solubility, dissolves more readily. In this case, the second step—permeation—becomes the rate-limiting factor. Even if we manipulate particle size, the overall permeation rate remains unchanged because dissolution is no longer the bottleneck. Therefore, particle size has a significant effect on anacetrapib but not on atazanavir.
How does particle concentration affect the particle drifting effect?
We observed that the unstirred water layer thickness increases as particle concentration increases. This means that although a higher particle concentration leads to greater permeability enhancement overall, the normalized enhancement per unit concentration decreases. In other words, the permeation process slows down at higher particle concentrations.
To understand this, we revisited the Whitney-Noyes equation. In addition to solubility, the bulk concentration within the unstirred water layer plays a crucial role. The dissolution rate is maximized when the bulk concentration is minimal, allowing for efficient drug release.
When more particles are introduced, the bulk concentration within the unstirred water layer increases, leading to "non-sink dissolution". This gradual slowdown in dissolution at higher particle concentrations results in reduced permeation efficiency, which explains the trend we observed in our data.
How do bile micelles influence drug diffusion and the particle drifting effect?
Bile micelles, endogenous surfactants in the gastrointestinal (GI) tract, play a key role in drug absorption and the food effect observed in poorly soluble drugs. We used sodium taurocholate as a model bile system and examined its impact on drug diffusion.
When we measured the unstirred water layer thickness in the presence of bile micelles, we found that for most drugs—danazol, efavirenz, estradiol, and felodipine—the micelle-bound drug either had a similar or thinner unstirred water layer compared to the free drug. This suggests that bile micelles can enhance drug diffusion, sometimes even more effectively than the free drug.
The effect of bile micelle concentration we observed is the opposite of drug nanoparticles. While increasing particle concentration led to a thicker unstirred water layer and slower permeation, increasing bile micelle concentration reduced the unstirred water layer thickness.
This is likely due to the unique mechanism of bile micelles—they move within the unstirred water layer, dissociate to release free drug molecules, and both the free drug and the micelle can permeate through the membrane. Since the free micelle itself is rapidly absorbed, it shifts the equilibrium toward more drug release into the unstirred water layer, enhancing the particle drifting effect. This explains why bile micelles improve drug absorption at higher concentrations and why the food effect is significant for poorly soluble drugs.
Pion Inc. provides an excellent platform for sharing cutting-edge research and fostering scientific discussions. It was a pleasure to present my work in collaboration with Pion, and I appreciate their commitment to advancing pharmaceutical science through knowledge exchange.
Watch Free Webinar: The particle drifting effect - a combined function of drug and colloidal properties.
About Prof. Na Li 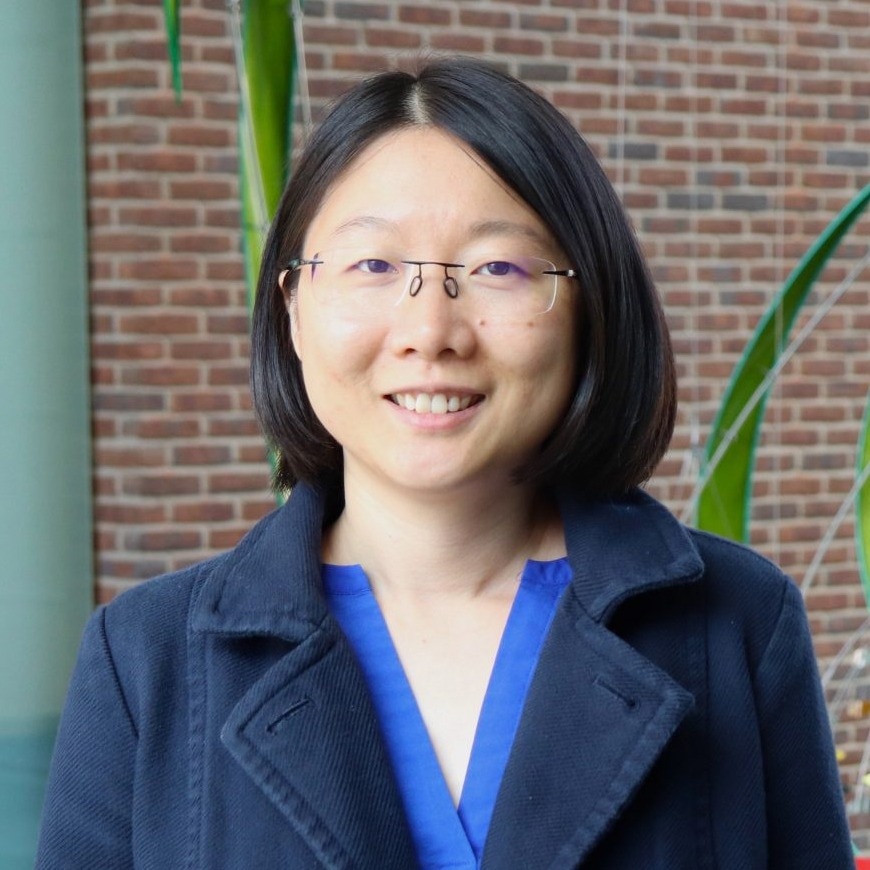
Prof. Na Li is an Assistant Professor in the Department of Pharmaceutical Sciences at the University of Connecticut (UConn). She earned her bachelor’s degree in Food Science and Engineering from South China University of Technology and her Ph.D. in Food Chemistry from Purdue University, followed by postdoctoral training in Industrial and Physical Pharmacy at Purdue. Before joining UConn, she worked at Crystal Pharmatech Inc., specializing in solid-state chemistry and crystal form selection of small-molecule drugs. Since establishing her lab in 2019, her research has focused on understanding the physical chemistry underlying interactions between formulations and the in vivo environment.
About Pion Inc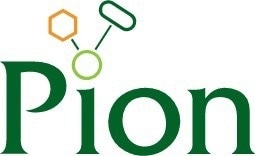
When data matters we apply out of the box problem solving abilities to help you reach a confident conclusion on your drug characterization challenges.
Pion supports the development of lifesaving and life-enhancing drugs by providing tools for drug developers, formulations scientists, and pharmaceutical production. For early-stage drug developers, our cutting-edge analytical technologies and services enable in vitro measurements of solubility, permeability, pKa and lipophilicity, providing essential data to improve candidate selection and formulations decisions for both oral and subcutaneous dosage forms. Later in development, high-pressure homogenizers enable particle size reduction and ensure material consistency from bench- to production-scale.