In this interview, Kevin Broadbelt of Thermo Fisher Scientific discusses the small molecule applications of process Raman spectroscopy.
How do cell therapies differ in complexity compared to monoclonal antibodies in terms of monitoring and process control?
Cell therapies introduce a heightened level of complexity due to the dual branches—gene-modified and non-gene-modified. For instance, gene-modified cell therapies like CAR T-cells involve specific viral vector manipulations. The challenge lies in monitoring these cells, which are crucial to the therapy's success.
Real-time monitoring of metabolites and critical process parameters (CPPs) becomes even more critical in this context because the cells act as vehicles for delivering the therapy. Monitoring factors like glucose levels become pivotal as fluctuations can directly affect product titer and even potential Post Translational Modifications (PTMs).
Downstream processing in cell therapies is intricate; while it can involve characterizing the product, monitoring for early detection of Critical Quality Attributes (CQAs) like aggregates is crucial. We use Raman spectroscopy to obtain quality information in real-time, considering both CQAs and CPPs.
Can you explain how Raman spectroscopy helps understand and control critical quality attributes (CQAs) in bioprocessing?
Raman spectroscopy is a potent tool in bioprocessing by offering insights into critical quality attributes (CQAs) at various stages. For instance, in upstream processing, it allows for real-time monitoring of metabolites.
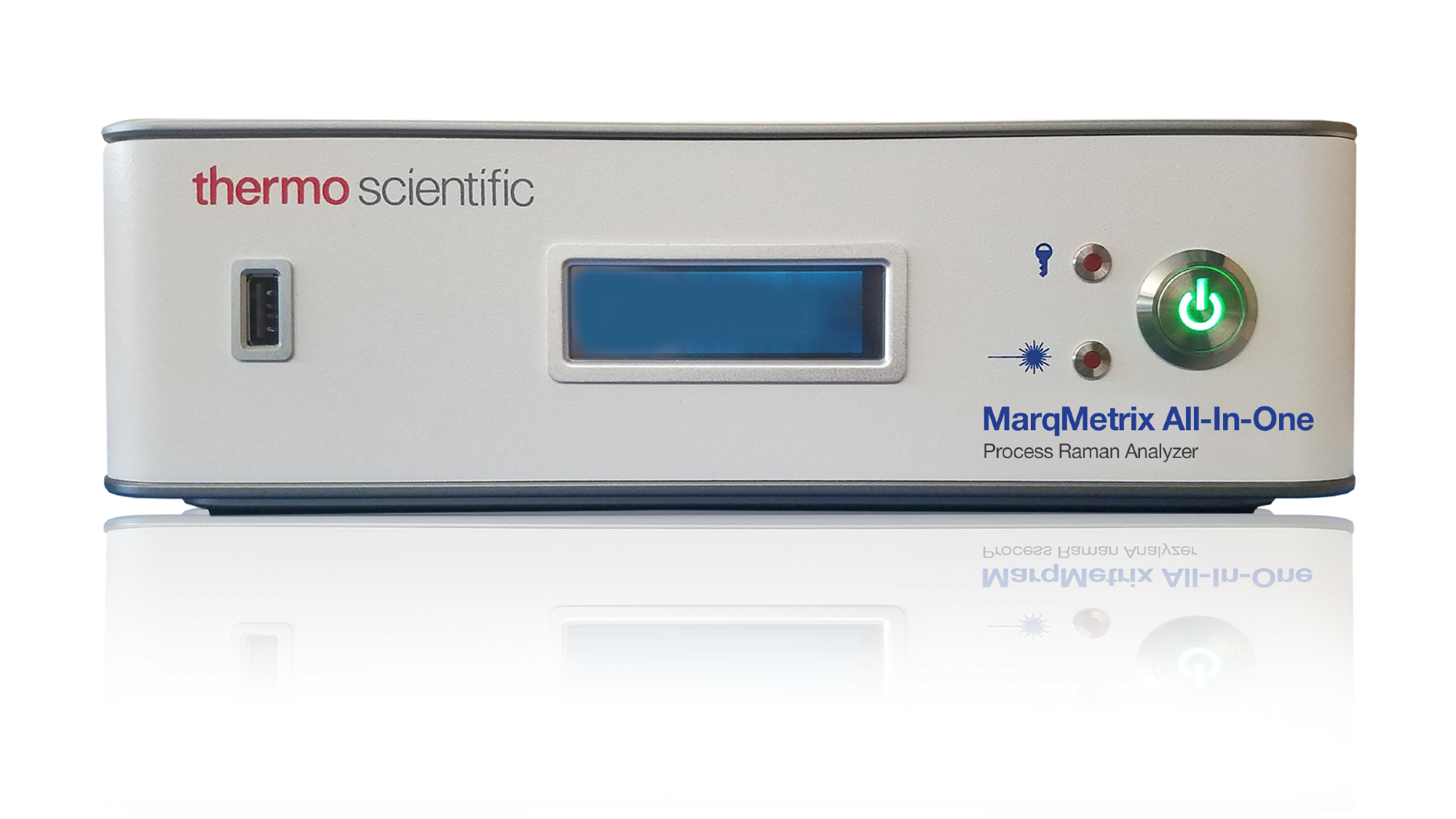
Image credit: Thermo Fisher Scientific
Glucose levels, vital for cell growth, can be monitored, ensuring that fluctuations are within a tight window to maintain product titer and avoid PTMs that could impact the product's functionality. Additionally, Raman spectroscopy's ability to monitor protein concentration accurately benefits downstream processes.
It enables precise quantification during ultrafiltration/diafiltration steps, crucial in maintaining protein quality and efficacy. Moreover, Raman spectroscopy's applicability across different modalities ensures its role in assessing specific markers or signatures, aiding in product characterization, and ensuring the product's viability and efficacy.
What challenges do stem cell therapies pose in terms of real-time monitoring, and how can process Raman spectroscopy address these challenges?
Stem cell therapies present unique challenges, particularly in the need for tight real-time monitoring across the process timeline. As stem cells transition from an adhesive state to a bioreactor environment, process Raman spectroscopy becomes instrumental in understanding these transitions.
It is crucial in tracking metabolites and CPPs, ensuring a comprehensive view of the cell's state during these transformations. Characterizing specific stem cell markers and understanding state changes within these cells is vital. Process Raman spectroscopy can aid in precisely identifying and characterizing these cells at different stages, allowing for a better understanding of their states and ensuring process control.
Stem cell therapeutics are still evolving, and employing Raman spectroscopy at various stages allows for an adaptive, dynamic approach to monitoring these crucial steps.
In what ways do mRNA therapeutics differ from monoclonal antibodies, and how does Raman spectroscopy address these differences?
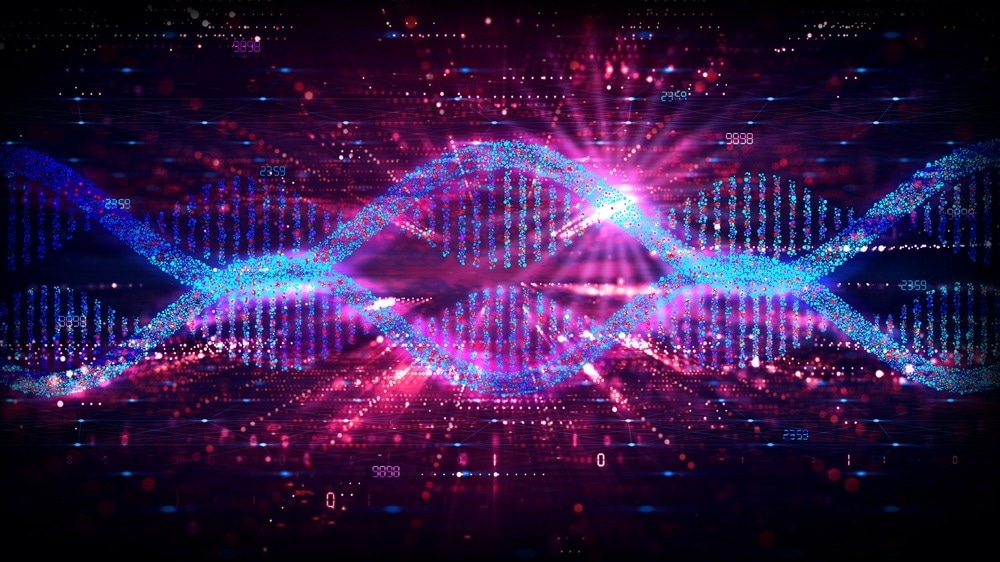
Image credit: ArtemisDiana/Shutterstock.com
mRNA therapeutics differ significantly from monoclonal antibodies (mAbs) in their production and monitoring processes. As a relatively unstable molecule, mRNA necessitates rigorous monitoring throughout its cell-free production stages.
Process Raman spectroscopy in mRNA therapeutics becomes pivotal, especially in monitoring the assembly of nucleoside triphosphates (NTPs), which form the mRNA product. Contamination, particularly double-stranded mRNA, poses a risk regarding immunogenic effects.
Raman spectroscopy allows in-process monitoring to detect contaminants, ensuring the final product's safety and efficacy. Raman spectroscopy's role also extends to post-purification stages, addressing contamination concerns and ensuring the quality of the mRNA therapeutic.
How does Raman spectroscopy contribute to accurate quantification during the ultrafiltration/diafiltration step in downstream processes?
In the downstream processes, specifically during the ultrafiltration/diafiltration step, Raman spectroscopy plays a crucial role in ensuring accurate quantification of protein concentration. Coupling the MarqMetrix All-In-One Process Analyzer with a FlowCell enabled in-line, real-time quantification of protein concentration.
The focus was on maintaining the quality and quantity of the protein product, which is essential for its efficacy. By employing acquisition parameters and meticulous testing for signal saturation across various concentrations of monoclonal antibodies, Raman spectroscopy provided precise quantification during the ultrafiltration/diafiltration process.
The impressive results show an excellent correlation between in-line Raman spectroscopy and off-line UV-Vis measurements for protein concentration. The percent error was notably below 5% across various concentrations, demonstrating the accuracy and reliability of Raman spectroscopy in quantifying protein concentration during downstream processes.
When implementing the process Raman spectroscopy for larger-scale bioreactors, what were the key observations and advantages compared to smaller-scale setups?
Transitioning process Raman spectroscopy to larger-scale bioreactors presented significant observations and advantages compared to smaller-scale setups. Several key insights emerged in an experiment coupling the MarqMetrix All-In-One Process Analyzer with a 500-liter Thermo Scientific™ HyPerforma™ DynaDrive™ Single-Use Bioreactor (S.U.B.)
The in-process monitoring involved around 20 measurements, considering acquisition time, laser settings, and data collection matching with off-line analysis. Chemometric modeling using independent data from multiple sets helped create accurate predictions for various metabolites.
The key observations centered around scalability and performance. Despite the larger scale, Raman spectroscopy maintained accuracy in predicting metabolite concentrations. It showcased robustness and reliability, with the model accurately predicting data points for metabolites like glucose, lactate, glutamine, viability, and titer.
The advantages were apparent in achieving consistent and accurate predictions across larger volumes. Raman spectroscopy demonstrated its scalability and transferability, proving its efficacy in optimizing and monitoring bioprocesses at larger scales without compromising precision or reliability.
Can you elaborate on the impact of fluorescence on Raman spectroscopy results and the methods used to mitigate this effect?
Fluorescence can significantly affect Raman spectroscopy results, posing a challenge due to its interference with the Raman signal. It arises when the sample emits light upon excitation, often overshadowing the Raman signal and complicating analysis.
To mitigate this, wavelength optimization of the excitation laser is a crucial step. Tuning the excitation laser to wavelengths like 785 or 1064 nanometers, away from common fluorescence peaks, can reduce the interference from fluorescence, allowing a clearer Raman signal to be detected.
Advanced pre-processing techniques are employed during data analysis to address fluorescence. These methods involve sophisticated algorithms to suppress or remove fluorescence contributions from the Raman spectra. By employing these techniques, analysts can isolate the Raman spectroscopy signature from the fluorescence background, enhancing the accuracy of spectral analysis.
How does Raman spectroscopy distinguish itself from Infrared Spectroscopy (IR)?
Raman spectroscopy and infrared spectroscopy (IR) both serve as valuable tools in analyzing biotherapeutics but have distinct differences. One significant contrast is their sensitivity to water-based media.
In the case of IR spectroscopy, the OH bond in water significantly affects the spectra, potentially dominating the peaks and complicating analysis in water-rich formulations. However, Raman spectroscopy has advantages in this context, as it is less sensitive to the OH group.
This distinction allows Raman spectroscopy to offer clearer and more interpretable spectra in water-based media, making it a preferred choice in biologics analysis where water-based formulations are prevalent.
What strategies can help circumvent routine calibrations to ensure uninterrupted operation during a run with multiple Raman spectroscopy systems?
Routine calibration can be a concern when utilizing multiple Raman spectroscopy systems, impacting operational continuity. Manufacturers are continually innovating to mitigate this issue. Some systems, like the MarqMetrix All-In-One Process Analyzer, employ factory calibration that significantly reduces or eliminates the need for routine calibration.
This innovation ensures longer uptime and minimizes interruptions during operation. Self-tests and validations are integrated into these systems to ensure reliability and robustness across instruments. The focus remains on achieving scalability without compromising calibration needs, addressing the downtime concerns associated with routine calibrations during a run.
How does monitoring IgG formation serve as a key process indicator in monoclonal antibody production, and how does Raman spectroscopy assist in this monitoring?
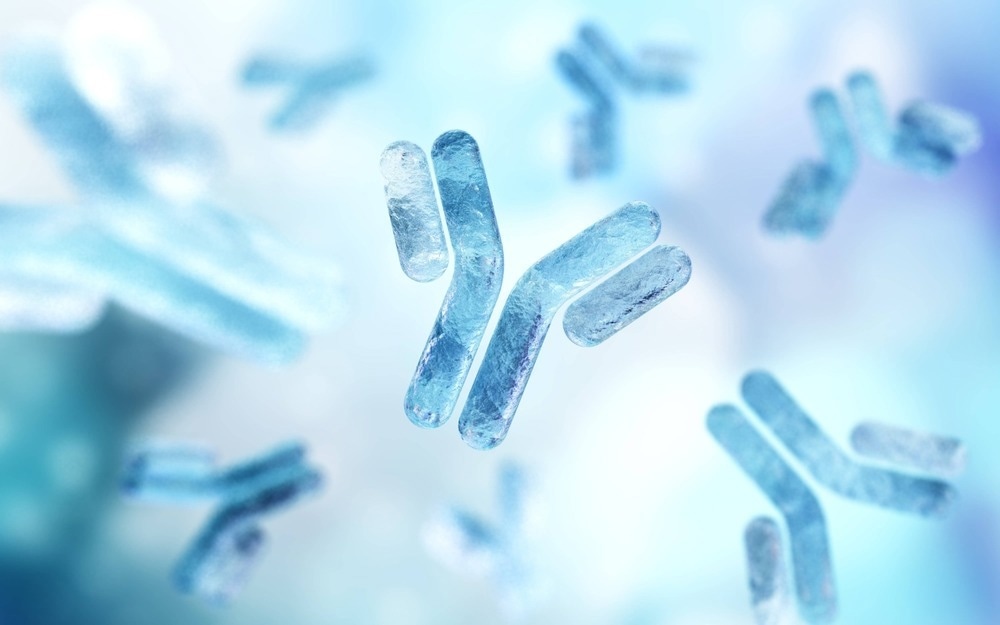
Image credit: ustas7777777/Shutterstock.com
IgG formation serves as a crucial process indicator in monoclonal antibody (mAb) production, directly linked to product titer and process performance. The IgG, produced by the cells, acts as a key process indicator (KPI), reflecting the efficiency and productivity of the production process. Raman spectroscopy contributes significantly to monitoring IgG formation during mAb production.
Raman spectroscopy enables real-time and non-destructive analysis of the cell culture media, allowing for the detection and quantification of IgG. By analyzing specific spectral patterns associated with IgG, Raman spectroscopy facilitates the continuous monitoring of IgG levels throughout the production process. These spectral fingerprints provide insights into the dynamics of IgG formation, offering crucial information on the overall progress and efficiency of the mAb production.
The ability of Raman spectroscopy to rapidly capture spectral data in situ without sample preparation or disruption empowers bioprocess engineers and researchers to closely monitor IgG formation, enabling prompt adjustments and optimization of the production process to ensure high product yields and quality.
What challenges are faced in gene therapy's upstream processing, and how does process Raman spectroscopy contribute to managing these challenges?
Gene therapy's upstream processing poses various challenges, notably in handling and monitoring the behavior of the cells used as vehicles for therapeutic delivery. Managing transfection efficiency, transvection complexities, and maintaining a conducive environment for cell growth are pivotal tasks in gene therapy.
Process Raman spectroscopy significantly contributes to managing these challenges by providing real-time insights into cell behavior and critical process parameters. It aids in monitoring the behavior of cells within bioreactors, assessing metabolites and crucial cell parameters essential for efficient gene therapy production.
Researchers can analyze metabolites, critical quality attributes (CQAs), and cell behavior in real time by employing Raman spectroscopy in upstream processing.
Raman spectroscopy facilitates the monitoring of viral vectors crucial for gene delivery. By examining spectral signatures associated with viral titers and vector characteristics, Raman spectroscopy provides insights into the efficiency and quality of vectors used in gene therapy.
How does Raman spectroscopy perform across different instruments or systems used in bioprocessing?
Raman spectroscopy showcases varying performance across different instruments or systems employed in bioprocessing. The key lies in its adaptability and the extent to which the instruments can be standardized for seamless transferability.
Instruments possess unique characteristics, especially when considering Raman spectroscopy, where certain systems might demand routine calibration. However, it is crucial to highlight that advancements in specific systems, such as the MarqMetrix All-In-One Process Analyzer, have shown promise by not necessitating frequent calibrations.
The calibration aspect is crucial in terms of scalability, ensuring that the models developed during process development remain robust and can be effectively transferred from small-scale to large-scale bioreactors. Transferability across instruments also involves accounting for diverse characteristics and ensuring that the models built for one system maintain accuracy when applied to others.
Ultimately, Raman spectroscopy's performance across instruments in bioprocessing relies heavily on the instrument's specifics, calibration needs, and the robustness of the methods developed for monitoring and controlling various parameters.
Considering the limitations and strengths, how can Raman spectroscopy best address the complexities of cell culture media in bioprocessing?
Raman spectroscopy is a valuable tool in navigating the intricacies presented by cell culture media in bioprocessing. One of the key hurdles lies in the formulation of the media, primarily water-based.
This composition often poses a challenge when employing specific spectroscopic techniques, such as infrared spectroscopy, due to their sensitivity to the OH group. However, Raman spectroscopy offers a distinct advantage by not being as susceptible to the dominance of water peaks in the spectra, enabling a more focused examination of the bioprocessing components.
The technique's strength lies in its ability to provide valuable information about various components within the complex media without being hindered by the strong water peaks. Despite the complexity of the media, Raman spectroscopy's robustness in handling such intricacies aids in precisely monitoring critical parameters and attributes within the bioprocess without interference from water-based components.
What data analysis methods or chemometric models have proven effective in interpreting Raman spectroscopy data for bioprocessing applications?
In the realm of bioprocessing, particularly in interpreting Raman spectroscopy data, successful methodologies often revolve around the application of chemometric models. These models are instrumental in extracting meaningful insights from the complex Raman spectra obtained during the process.
Chemometric models aid in processing and analyzing the extensive spectral data generated, allowing for the identification and quantification of various metabolites, critical quality attributes (CQAs), and process parameters. Key methods encompass sophisticated chemometric modeling techniques, including multivariate analysis and machine learning algorithms.
These methods involve preprocessing steps and robust model development strategies to effectively discern relevant information and trends from the spectra. Furthermore, these chemometric models are tailored to capture and interpret the spectral fingerprints unique to different bioprocessing stages, enabling a comprehensive understanding of the process dynamics and aiding in real-time monitoring and control.
What is an example that illustrates the comprehensive role of process Raman spectroscopy in ensuring high-quality biological product quantity and performance?
Let us consider a practical example of a well-established workflow for monoclonal antibody (mAb) production. In this case, the MarqMetrix All-In-One Process Analyzer was integrated into a typical 14-day run with a five-liter bioreactor. The aim was to showcase the comprehensive role of process Raman spectroscopy in ensuring the biological product's quantity and quality.
The MarqMetrix All-In-One Process Analyzer was utilized for in-line monitoring of metabolites, including glucose, glutamate, glutamine, viability, and titer. Through the integration of chemometric modeling, we optimized cross-validation from both reactors and compared the outcomes to offline data.
The observed results demonstrated an excellent correlation between measured and predicted concentrations of crucial metabolites such as glucose, glutamate, and lactate. Moreover, viability and titer, key indicators of cell health and product yield, also showed highly correlated results.
This example highlights the power of process Raman spectroscopy in providing a wealth of real-time data, ensuring robust and efficient manufacturing control, and ultimately contributing to producing high-quality biologic products.
About Kevin Broadbelt
Kevin Broadbelt is a Global Bio/Pharma Applications Scientist in Strategic Marketing with the Field Safety Instruments at Thermo Fisher Scientific. He completed his postdoctoral work at Boston Children’s Hospital & Harvard Medical School focusing on neuropharmacological studies and proteomics-based candidate biomarker research. He completed his Ph.D. at the University Center of the City University New York in 2006. Kevin is also an active volunteer for MassBioEd Life Sciences Apprenticeship Program, past lecturer for APEC Biotherapeutics Center for Excellence at Northeastern University and past director of the Biotechnology & Bioinformatics.
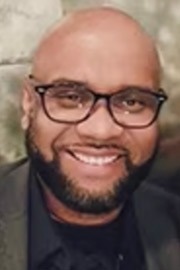
About Thermo Fisher Scientific – Materials & Structural Analysis
Thermo Fisher Materials and Structural Analysis products give you outstanding capabilities in materials science research and development. Driving innovation and productivity, their portfolio of scientific instruments enable the design, characterization and lab-to-production scale of materials used throughout industry.
X-ray fluorescence spectrometers, FTIR spectrometers and X-ray photoelectron spectrometers enable researchers and material analysts to probe the chemical composition of materials ranging from ultra thin-films to bulk powders used in product development and materials troubleshooting. We offer a line of rheometry and viscometry instruments, as well as single- and twin-screw extruders, for laboratory benches that enable you to measure the physical property of semi-solids and liquids for shear flow and yield stress.
No other company offers the breadth of products and the depth of analytical capabilities for materials science and engineering.