Please can you give an overview of your research interests?
In my lab, we focus on understanding structure, assembly and regulation of the LC8 protein interaction network, the array of LC8 interactions with diverse partners which affect multiple cellular functions in biomedical systems.
Most proteins are involved in interactions with one or a few functional ligands, but some proteins, like light chain 8 (LC8), have numerous binding partners. LC8 interacts with more than 100 functionally different proteins, from viral proteins to motor proteins to proteins involved in cell division.
LC8-IDP Interactions | Protein NMR in a New Era of Biomedical Research
LC8-IDP Interactions | Protein NMR in a New Era of Biomedical Research from AZoNetwork on Vimeo.
While LC8-binding proteins have many different functions, we've proposed that they all share a common feature, namely, all are members of a relatively new class of proteins, often called intrinsically disordered proteins or IDPs, that lack – in whole or in part – a stable tertiary structure. IDPs do not fold into a tightly packed native state that presents one or a few specifically arranged active sites for binding of a protein or other substrate. Instead, IDPs sample multiple inter-converting conformations that have varying degrees of disorder, and a subset of these can bind specifically to several other folded proteins.
The intrinsic, or inherent, attributes of IDPs are that they are disordered before they bind a partner protein, they retain some degree of disorder after they bind, and disorder is an important aspect of their function. What is special about LC8, a protein that is essential to the life of the cell, is that it binds disordered proteins and mediates their dimerization. Being a dimer itself, LC8 binds two chains of a disordered protein and brings them together to form a duplex scaffold onto which other proteins assemble with higher affinity than when they bind a monomeric IDP.
How important is NMR in your work, and what benefits does it provide over other analytical techniques?
To characterize important features of an LC8-IDP duplex, we need an atomic level description of its structure. The techniques available for characterization of high resolution structure such as X-ray crystallography or cryo-electron microscopy require a stable protein with a unique conformation. They are not suited to characterization of complexes involving intrinsically disordered proteins. For our studies, NMR is superior to the other techniques because NMR monitors regions of disorder in large multimolecular complexes.
We can identify and characterize various conformations sampled in disordered proteins and identify conformational changes that accompany binding, both at the site of binding and, more importantly, at sites distant from binding. In LC8-IDP duplex scaffolds, some disordered regions form higher order structure, and we can identify those regions by NMR.
Please can you give an example of your research into an LC8 partner?
One LC8 partner is dynein intermediate chain (IC), a protein from the dynein motor, a large complex whose role in the cell is to move molecular cargo from one place to another. There has been fascinating research done on how this complex moves along microtubules. But we are interested in a different region of dynein, the one that latches onto cargo – we ask what determines where cargo binds to the complex, what regulates when this cargo moves and finally how/why the complex releases the cargo when it reaches its destination?
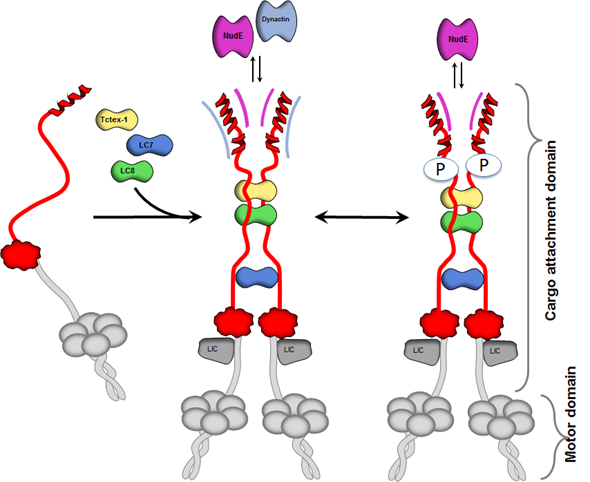
An interesting aspect of this process is that there are additional proteins that bind dynein and direct, or regulate, cargo binding and, like LC8, these proteins bind to a disordered IC region, making it a hub of cargo regulatory activity. This is where NMR comes in – this disordered region can only be studied using NMR. When a regulatory protein binds the complex, we use NMR not only to identify specific regions of a disordered protein that interact with the regulatory protein, but also to monitor conformational changes in regions that retain disorder in the complex.
Thus, the disordered region is important because this is where regulators bind dynein and tell it what cargo to carry and when to move it. Among the regulators that bind this domain are proteins associated with Huntington's disease or mental retardation, systems with important biomedical implications.
What has your research shown?
We showed that a key domain of dynein IC that is central to dynein function is intrinsically disordered. This large domain of about 300 amino acids is the site of dynein intermediate chain/light chain assembly, and the region to which many cargo regulating proteins bind.
We showed cases where binding at one site in the long disordered chain alters binding of a regulator protein at a distant site on the same disordered chain. One change in the amino acid sequence in one disordered region can determine whether a protein binds or does not bind to a completely different disordered region. Thus, one genetic mutation that affects the protein sequence in a disordered region can affect binding at a distant site, and make a qualitative difference in cellular function.
This may be the molecular basis of some diseases. We perform NMR experiments that tell us which specific amino acid residues, or mutations, are associated with an essential cell function. If a disease is traced back to the loss of that cell function, this molecular structural knowledge may lead to a treatment of the disease.
Another interesting feature of disorder in dynein intermediate chain is its effect on tissue-specific differences such that the same protein works differently in the brain than in muscles. The only difference between brain vs. muscle IC is the length of disordered linkers. To determine why a small increase in the length of a disordered linker determines what happens in the brain relative to what happens in muscles tissues, we require atomic level techniques.
Our data imply that changing the length of a disordered region can have a big effect on the population of transient structures that determine how this protein behaves, although the sequence of the rest of the disordered protein hasn't been altered. Even though there are recent beautiful X-ray and cryo electron microscopy structures of this complex, they do not answer this question because disordered structure is not evident in these images.
Disordered linkers are also the sites of phosphorylation events which determine the function of the proteins. We've shown that phosphorylation in a disordered linker can affect binding at a distant disordered site by changing the conformations of a family of transient, interconverting structures. We don't see ‘on-or-off’ conformations but rather a dynamic shift in an array of related conformations. This is a type of structural biology we can observe only with NMR.
How were these complexes traditionally studied and why is NMR so useful in your studies?
These large complexes have traditionally been studied by X-ray crystallography or cryo-electron microscopy but regions of disorder are silent in crystallography. Because it's disordered, we see everything except this region, a problem if this is where we want to study molecular activity.
This illustrates why NMR is useful: disorder limits the use of electron microscopy or crystallography, but can be characterized using NMR. You can identify the sequences that are disordered, then determine exactly which disordered amino acids bind another protein and when/why one regulator binds while another does not.
For example, in a virus protein with a disordered region that binds LC8, if LC8 does not bind this protein, the virus loses its ability to produce disease-causing infections. NMR can identify binding sites in these regions and potentially identify drugs that interfere with the LC8-virus protein interaction.
So far I've talked about how beautiful NMR is and how it's the technique that reports changes in specific amino acid residues in disordered proteins. While this is true, there are quite a few challenges to using NMR on disordered proteins, compared with folded proteins. With disordered proteins, you notice very limited peak dispersion in NMR spectra. You also have multiple conformations that are difficult to sort out and that can result in exchange broadening, meaning peaks disappear in the spectrum. But NMR peak broadening before disappearance can also tell us about the conformational properties of disordered regions as they begin to interact with LC8 and other proteins.
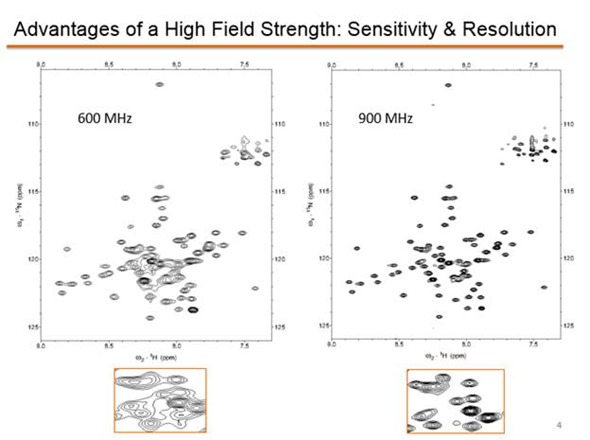
How important is basic science to creating treatments for diseases? Does your research focus on any particular disease?
We focus on the basic science that identifies molecular processes that have an essential function in cells. Our goal is to understand fundamental cellular functions of LC8-IDP protein complexes in biomedical systems. Then we can apply what we understand in a broader arena that includes how a disease disrupts the fundamental functions of LC8-IDP duplexes. This in turn can lead to how this disease might by treated by drugs that prevent the disruption.
Several diseases tie into our studies of the disordered dynein IC where regulators bind dynein and tell it what cargo to carry and when to move it. Among the regulators that bind this domain are the proteins associated with Huntington's disease or mental retardation.
We study other LC8-IDP duplexes, not associated with dynein, that are also involved in interactions with a lot of proteins. Examples include the nuclear pores, which require LC8 for their assembly and some viral infections such as Ebola and rabies virus.
For example, in rabies virus there's an LC8-IDP interaction we are looking at now with a virus protein. The interaction of this protein with LC8 makes the difference between whether mice infected with the virus live or die. One small but crucial change in that interaction determines whether the virus is lethal in mammals.
What are your thoughts on funding for NMR in the US and Europe?
During a year on sabbatical in Germany and France, I was very fortunate to have access to super high field 900+ NMRs. I was surprised at how much funding there is for NMR instrumentation in Europe.
In Europe they don’t have an instrument in every lab but rather have instruments in central facilities. These facilities have managers who reach out to users and provide help and expertise, allowing people who may not normally have access to NMR the ability to use the instrument.
Also, they are very good in outreach activities to communities and high schools, making them aware of the importance of basic science. I've reviewed proposals by European investigators that propose basic science experiments without having to link it to disease because basic science is considered important enough.
In the US, it seems there is more push to link research to a disease cure rather than to furthering our understanding of the basic science that serves as the foundation for development of cures. And we lack the types of centralized facilities Europe has. I was pleased to lead efforts to attract federal funding for an 800 MHz here at Oregon State by creating a facility that will service researchers in our region.
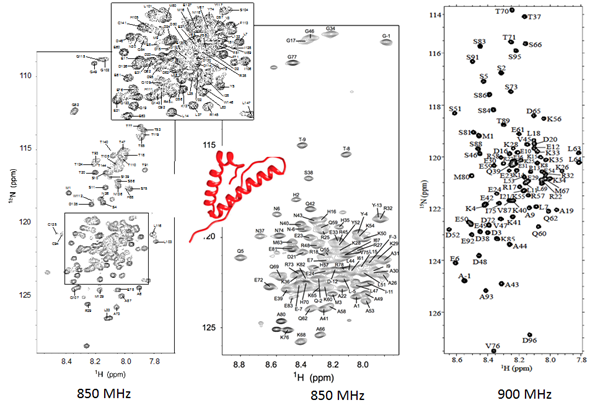
Many researchers want to use NMR once they have access to an instrument, qualified people running it and people like me who are enthusiastic about making it accessible. When this happens, the power of NMR becomes apparent to researchers in many disciplines.
What do you think the future holds for protein research using NMR?
I think these are very exciting times for NMR and NMR-based research on disordered proteins. We try to push the envelope in the kinds of questions we ask regarding large, complex and dynamic protein assemblies. We ask questions that a few years ago were only a dream because of the difficulty of working with disordered protein complexes.
Now, we have considerable advancements in higher fields, cryoprobes that allow data collection on dilute samples, pulse sequences that allow data collection in five dimensions (and so decrease peak overlap) and computational techniques that make use of only sparse NMR data.
Along with NMR advancements, we have new creative approaches to the routine preparation of complexes of disordered proteins, and enhanced multidisciplinary approaches. Together this positions us to make these dreams more of a reality.
In summary, NMR is a powerful technique because it can monitor in large disordered complexes regions of disorder and regions of interaction, and identify which amino acids are involved in interactions. That information can be used to identify changes in structure at the site of binding and also at a distant site and whether there is self-association. You cannot get this information about disordered proteins using any other technique.
About Elisar Barbar
I'm a professor in the Department of Biochemistry and Biophysics at Oregon State University and my primary tool is NMR. The research in my lab is focused on large macromolecular complexes organized around a unique type of scaffold first described by us, a duplex formed by the association of the folded protein LC8 with two chains of a fully or partially disordered protein.
In demonstrating that an LC8-containing duplex scaffold is a central component of numerous essential cellular systems, we applied NMR techniques to elucidation of the roles of protein disorder in cell function.
I am pleased to lead the recent successful efforts at Oregon State University for funding of major instrumentation grants for a multi-user 800 MHz NMR. The new instrument will be the highest field NMR in the state of Oregon, will support other universities in the region, stimulate new collaborative projects, and, most crucially, will allow us to keep developing innovative approaches towards revealing how dynamic large protein complexes work.
About Bruker
Bruker is market leader in analytical magnetic resonance instruments including NMR, EPR and preclinical magnetic resonance imaging (MRI). Bruker's product portfolio in the field of magnetic resonance includes NMR, preclinical MRI ,EPR and Time-Domain (TD) NMR. In addition.
Bruker delivers the world's most comprehensive range of research tools enabling life science, materials science, analytical chemistry, process control and clinical research. Bruker is also the leading superconductor magnet and ultra high field magnet manufacturer for NMR and MRI solutions.