In this interview, Karen Esmonde-White from Kaiser Optical Systems talks to News-Medical Life Sciences about measuring Salmon Meat composition using Near-infrared Raman Spectroscopic techniques.
What are the main components in salmon that an analytical tool would need to measure during processing?
We all know that salmon is a popular dish and can be a very tasty food. We also know that there is high consumer demand for salmon worldwide. This demand creates a need for efficiency from the initial raising of the salmon to the point when it is processed in the plant. Rapid measurement of quality can help achieve this goal.
Ideally, you would want your analytical tool to predict the three main attributes of meat quality: fat, color, and texture. In addition, the analytical tool would need to be rapid, non-destructive, and robust to the plant environment, whether you are measuring before the slaughter or in the processing plant itself.
For maximum efficiency, the primary three measurement components of salmon could be measured using a single probe because you do not want to be stopping the plant to take a measurement three times. To do so, you would need the tool to be hygienic, or to not come in direct contact with the tissue.
Tests for these three components are most often performed in the laboratory. For fat content, people use the American Oil Chemists' Society method to examine the fat saturation, otherwise known as the iodine value. Newer methods may use liquid or gas chromatography.
We can also these principles of lab measurement when it comes to determining color. For color identification, you look at the Roche color card or do a carotenoid extraction in the laboratory and use chromatography or reflectance (which is UV-Vis based). For firmness, you are doing standard mechanical testing by looking at the mechanical deformation or doing extractions for collagen or moisture content.
These laboratory-based techniques are not efficient, and most importantly, they are destructive. You need to take a sample out of the processing plant, bring it to the lab, perform your reaction, and then wait. Such inefficiency does not yield real-time measurement. Simply put, salmon’s higher product demand is driving industry for tests that are non-destructive, automated, and inline.
To get inline measurements, the field is looking at various optical and spectroscopy technologies. These include UV-Vis, hyperspectral imaging, machine vision (a high-speed camera with automated color), near-infrared for moisture, and Raman spectroscopy.
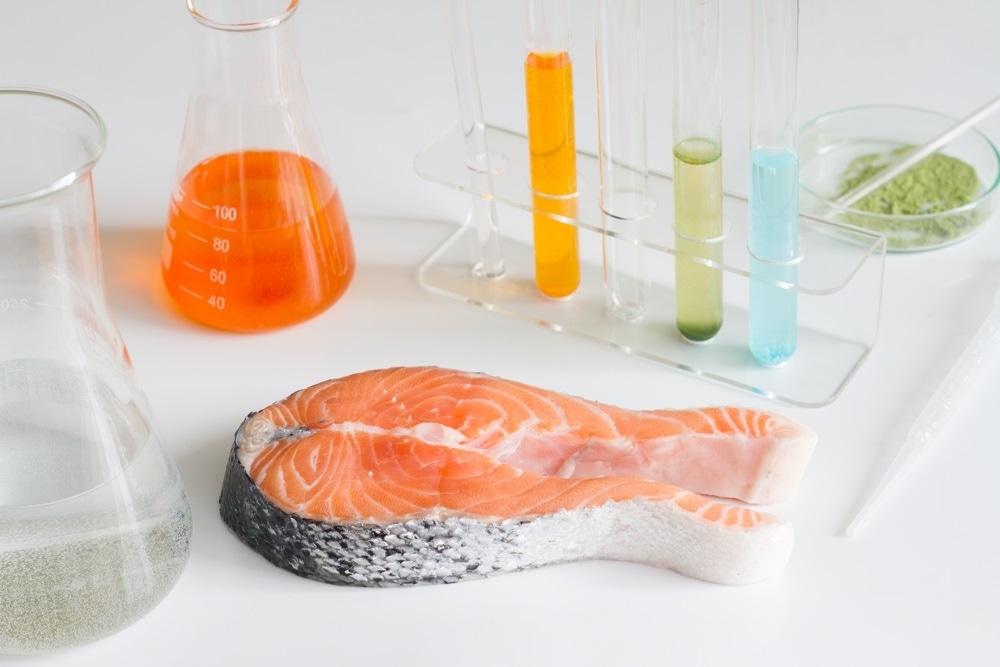
Image Credit:Shutterstock/udra11
What does Raman spectroscopy measure? How does this analysis technique benefit the analysis of salmon products?
Raman spectroscopy measures scattered light. It uses a laser that will illuminate the sample. Most of the scattered light will not change in wavelength, so it will return as the same color. However, one out of every million photons that laser light will interact with causes molecular vibration (making Raman a one in a million phenomenon). In these cases, it will lose a little bit of energy, and we can measure that change of energy.
That change of energy is based on the functional groups or the chemistry of the material that we are examining. The result is a spectrum, and sometimes we call that spectrum a molecular fingerprint because it gives us very specific information on the chemistry of the material.
Because it uses visible or near-infrared light, the advantage of Raman spectroscopy is that we can use visible optics that do not require specialty materials, which provides a great deal of sampling flexibility. We can measure solids, liquids, gases, and we can do this inline or in the laboratory.
We can use Raman in three different ways. First and foremost, it can chemically identify what we are looking at. For example, we can identify that our sample is a pharmaceutical small molecule and it is in crystal form. The Raman spectra does an excellent job of differentiating between crystal forms. The second use is for quantitative purposes. For example, we can measure glucose solutions in a sample and are able to measure from 0.5 grams per litre up to 15 grams per litre.
In addition, the identification and quantitative measurements can be integrated to understand whether the molecular fingerprint has changed, and if so, by how much during a process. One very powerful application of this is identifying the endpoint of a reaction based on the disappearance of our reactants and the formation of our products.
Other advantages of Raman spectroscopy are that it provides direct, real-time, and in-process measurement. Some Raman systems are compatible with hazardous area environments such as petrochemical fields and chemical plants. The analyser can “talk” to a control room via industrial communication platforms, thereby avoiding the need for manual user intervention.
With Raman spectroscopy, we can measure the absorption, refraction, and scattering. Some materials have multiple interactions with the same matter, which is certainly true for biological tissues including meat and fish products. The reason why spectroscopy is so powerful is that it can examine the materials' chemical properties non-destructively based on changes in transmission, absorption, or wavelength. Raman spectroscopy is a powerful technique because we can understand the chemistry of something in real-time without needing to prepare or destroy it.
Your background is in biomedical and tissue optics. Did your work in this field relate to your experiments with salmon tissue analysis?
Yes. As I am looking at Raman spectroscopy of salmon, I look at it as a food. However, scientifically, I can also look at it as a biological tissue and apply my training in biomedical optics. Biomedical optics is the field in which we are developing spectroscopy for measuring biochemical changes of tissues in health, aging, and disease, with the aim of clinical measurements being to observe these biochemical changes that may precede or accompany disease.
Tying the principles of Raman spectroscopy with tissue optics results is the field of tissue Raman spectroscopy. Similar to spectroscopy, we do tissue optics every day. We see it when we go to see the doctor for a blood oxygen measurement, for example. That is biomedical optics in action. We can also see some principles behind biomedical optics when we put a laser on our finger. Our finger glows instead of the laser just going straight through our finger. We might be able to also see some changes of intensity as well.
While the field of tissue Raman spectroscopy has been around for a long time, it was advanced in the early 2000s when Mike Morris and Pavel Matousek were first able to demonstrate that they could measure subsurface Raman spectra. Bringing in some principles from tissue Raman spectroscopy, such as understanding sampling volume and incorporating the use of tissue phantoms, can help us build a comprehensive approach to meat quality measurements using Raman.
This understanding happens because we have heterogeneous tissues that can absorb light and bounce light around. Consequently, we see changes in absorption or scattering. We can also see that light is sometimes just reflected back and it just goes through the tissue very, very rapidly. All these things are happening at once, and we can understand those complex interactions in the laboratory.
An important tool we have in the laboratory are specially designed physical models, also called tissue phantoms. They are not scary! Tissue phantoms are used in hospitals and medical facilities every day to calibrate our medical imaging equipment such as X-rays, CT, MRI, and PET equipment. They are also used for the biomedical optics community because they allow us to understand instrument performance, and they can also be very useful in food analysis.
Using these well-defined models is especially important for chemical analysis such as Raman spectroscopy. While we can go to the store, or we can work with a processing plant to get specimens, we cannot define the composition precisely. What a tissue phantom allows us to do is build something in the laboratory very easily, very simply, and very quickly with a precisely defined composition.
Using tissue phantoms has several benefits including optimising what probe to use, understanding measurement performance, and serving as starter data for our analytical model. What is interesting about tissue phantoms is that because you are making these in the laboratory, you can define the zonal variations too.
For example, for the red portion phantom, you can make distinct fat inclusions in a layer of muscle. That can mimic the red portion, and it is easy to define the percentage of fat as well. For a white portion phantom, you would want to have something where you do not have the very distinct zonal variations. For this, you would probably want to mix your zones a little bit more, and that is something that you can easily do with a tissue phantom.
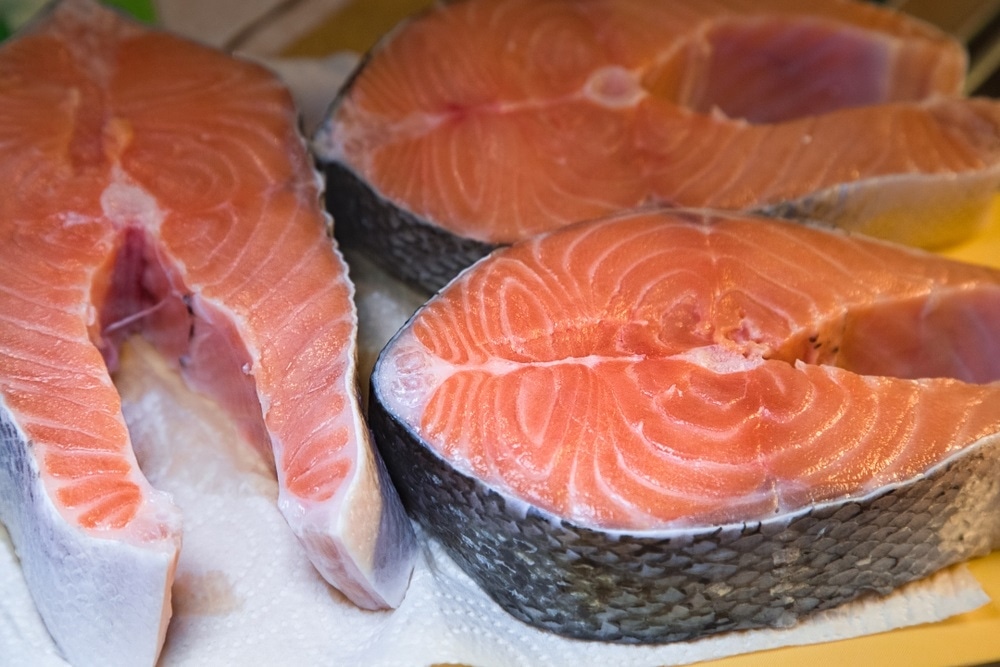
Image Credit:Shutterstock/Milovzorova Elena
Are the common Raman spectroscopy wavelengths of 785 nm and 1000 nm useful for the analysis of food products?
In Raman spectroscopy, we talk about using 785 nm or even 1000 nm as a way to reduce fluorescence. That is one reason that we use those wavelengths. In tissue measurements, we have additional benefits of going to these wavelengths. These include balancing the effects of water or haemoglobin absorption at different wavelengths, or trying to optimize for one or the other, which is a really important component of tissue optics.
Could you give us more detail on the experiments you conducted to analyze salmon samples?
We performed two studies. The first study was to assess the utility of the 1000 nm system for measuring fat, color, and texture components in salmon tissue. Salmon Raman spectroscopy has been around for at least 15 years, but it had all been done at 785 nm and never at 1000 nm. We had the 1000 nm system and wanted to know what would happen if we used this wavelength. The second study was to assess the compatibility of an offset non-contact probe at 785 nm for inline meat quality measurements. For both studies, we simply used store-bought samples. My colleague had gone to our local grocery store and just picked up every type of salmon specimen that she could find, including some farmed Atlantic salmon, wild coho salmon, and smoked salmon.
We measured it in as many ways that we could in the laboratory. We measured the meat in various locations and we also collected on the skin. We took measurements on dark and light skin using a contact probe and non-contact optic. We were able to collect good spectra within one and ten seconds. We used the Raman Rxn2 analyzer that operates either at 1000 nm or 785 nm, and the laser intensity was about 80 milliwatts.
We used a contact optic, so we were able to get surface measurements of the solids when we were able to touch the probe to those different sections. These probes use the backscatter geometry. The illumination and the collection fibres are close together, and it gives a small spot size that provides surface measurement. With the contact probe, we were able to see the zonal heterogeneity. We could look at the fat section versus the muscle section, and get information on just the fat, or information on just the muscle.
The non-contact optic uses a similar backscattered Raman approach and it provides a small spot size, but it is non-contact. For the inline process probe, we used our non-contact PhAT probe. It uses a large volumetric approach to collect surface and subsurface Raman signal. This approach is especially useful in processes involving solids for a couple of reasons. The first reason is to obtain a large volume measurement that is representative of the sample quickly with a single measurement. The second reason is that we can do this without needing to focus the probe onto the sample for every new sample.
What were the results of these experiments?
First, we needed to get the meaning from the spectra we generated. The different bands that we saw corresponded to different attributes in the tissue. We observed bands that corresponded to collagen, fats, proteins, and the carotenoid pigments. These bands have been shown to inform on the three major components of fish quality—firmness, fats, and color. So, we were able to get a multi-attribute measurement with just a single probe and a single measurement.
The use of 1000 nm had its advantages. It did give us high spectral resolution and less water absorption. I would say that in terms of reducing fluorescence, it did reduce fluorescence slightly. I am really pleased with the 1000nm system because it was able to provide spectra rapidly that are consistent with literature reports on salmon meat proteins, fats, and carotenoids. Using the 1000 nm system with a small spot size probe allowed us to see the compositional heterogeneity in salmon meat. The PhAT process Raman probe is compatible with the cycle time of the processing plant for inline meat quality measurements.
About Dr. Karen Esmonde-White
Dr. Karen Esmonde-White is a Senior Marcom Specialist at Kaiser Optical Systems Inc.
-2.jpg)
She earned her B.S. in chemistry from Wheeling Jesuit University, her M.S. in Chemistry, M.Eng. in Pharmaceutical Engineering and Ph.D. in Biomedical Engineering from the University of Michigan. Dr. Esmonde-White has over 6 years experience as an analytical chemist in the pharmaceutical industry.
Her research interests are in biological Raman spectroscopy, process analytics and instrument design.