The underlying principles of Raman spectroscopy and the components that make up a complete Raman Spectroscopy solution are outlined in this article.
Understanding the Raman effect
The Raman effect is based on light scattering. In most instances, light that “hits” a molecule does not interact with it and so the photon does not lose energy. This process is known as elastic scattering, which is the phenomenon responsible for your finger appearing to glow when you hold a flashlight onto the tip of it.
Yet, in one out of around 1,000,000 scattered photons is scattered inelastically: this means that the photon does interact with a molecule’s vibration or rotation. The photon loses a small amount of its energy to the molecule because of that interaction, resulting in a wavelength that is slightly increased.
From the side of the molecule, when a molecule’s vibration “absorbs” energy from a photon in the visible, ultraviolet or infrared range, that photon’s energy excites the vibrational energy in the molecule’s chemical bonds. This is known as the Raman effect.1
This loss in photon energy is identified as a slight difference in frequency between the scattered and the original photon, corresponding to a specific vibrational mode of the chosen molecule. The amount of energy transferred is so specific that measuring the frequency shift of these one in a million photons allows the accurate identification of that molecule.
This is the basis of Raman spectroscopy: to establish which compounds are present, a mixture of molecules is illuminated with monochromatic light (usually in the form of a laser), and the frequency shift of the inelastically scattered photons is measured.
Capturing and processing Raman data
A Raman system will typically observe backscattered photons, which means that the probe that delivers the laser light to the sample also captures the photons emitted back towards it.
An optical notch filter is utilized to attenuate photons of the same frequency as the laser so that only the frequency-shifted photons remain. This is done to isolate the Raman photons.
Like a glass prism, this light then passes into a spectrometer, where it is “split” into its different frequencies. The intensities of each frequency are then measured. Actual detection of the photons takes place on a CCD sensor.
A Raman spectrum is the result of all of this and can be visualized as a plot of intensity against frequency. Each Raman-active molecule has its own unique “fingerprint”, which is exhibited as peaks in this spectrum. Raman spectra typically feature large numbers of sharp peaks, which allow the identification of molecules even in complex mixtures.
Raman vs other spectroscopy
Raman spectroscopy is uniquely powerful due to the physical nature of the Raman effect. The benefits of Raman are best understood in comparison to other popular light spectroscopy methods such as Fourier-transform infrared spectroscopy (FTIR).
FTIR is widely utilized throughout the biosciences and physical sciences. FTIR involves exposing a sample to a range of frequencies and measuring the relative absorption of each frequency, unlike Raman, where a sample is illuminated by monochromatic laser light.
FTIR creates a spectrum which, broadly speaking, is seen in a similar way to a Raman spectrum: certain absorption “peaks” correspond to certain energy transitions that can happen in a particular molecule, which, in turn, can be employed to identify compounds.
A comparison of a Raman spectrum and an FTIR spectrum of the same molecule exhibits some differences. Peaks that show strongly on a Raman spectrum will show weakly on an FTIR spectrum. Raman and FTIR (or mid-IR) can be considered as complementary methods – each spectroscopy method has its own sensitivity to different molecules.
One of the benefits of Raman is that it is relatively insensitive to water. This means that it is especially well-suited to measuring the constituent of aqueous solutions. Furthermore, Raman is ideal for the analysis of inorganic compounds, in addition to organics, opening a wide scope of applications beyond those of FTIR.
Sample preparation time is another benefit. Where FTIR and several other spectroscopy methods need manual sample preparation, Raman usually (though not always) does not need any.
Typically, setup is as easy as focusing a probe on a sample. Next, measurements can be taken automatically and frequently, providing an essentially real-time analysis which allows measurement of the evolution of reactions as they happen.
Maybe the biggest advantage of Raman is that it is quantitative: it can tell us the relative proportion of each chemical species present in a sample because the Raman effect scales linearly with the amount of a substance present.
Components of a Raman system
There are three fundamental components in a Raman system: probe, analyzer, and software. To produce unparalleled results, Kaiser Optical Systems have developed unique approaches to each of these components.
The probe is the part of the system that interfaces with the sample/process being measured. Kaiser Optical Systems provides a wide range of sample-type specific probes that will suit any application.
For instance, the PhAT probe supplies an ultra-wide measurement area while eliminating the requirement for aligning the probe for surface roughness. This is ideal for solids.4 The AirHead probe is a combination probe and optic combination built for gas phase analysis.5
A variety of compatible optics ensure that Kaiser Raman systems can collect accurate measurements of any type of sample. For example, the bIO-Optic is optimized for benchtop bioreactor applications requiring head plate entry.6
The heart of a Raman system is the analyzer, which contains the laser to generate the incident light, and the camera which analyzes emitted light. Kaiser Optical Systems provides two analyzers: the Raman Rxn2 and Raman Rxn4.2,3 Essentially, these models have different enclosures to suit different process environments but possess the same high-performance core components.
The system’s software controls the components in the analyzer and generates spectra from the information gathered by the CCD. Kaiser Optical Systems developed Raman RunTime, which controls the analyzer and hosts a Raman model to enable real-time analysis.
Bringing these components together can create Raman systems that possess a wide range of capabilities.
References and further reading
- Symmetry and Spectroscopy: An Introduction to Vibrational and Electronic ... - Daniel C. Harris, Michael D. Bertolucci
- Kaiser Raman Rxn4 | Kaiser Optical Systems, Inc. Available at: https://www.endress.com/en. (Accessed: 21st August 2020)
- Kaiser Raman Rxn2 Analyzer from Kaiser Optical Systems, Inc. Available at: https://www.endress.com/en. (Accessed: 21st August 2020)
- Kaiser Raman Phat Probe | Kaiser Optical Systems, Inc. Available at: https://www.endress.com/en. (Accessed: 21st August 2020)
- Kaiser Raman AirHead Probe | Kaiser Optical Systems, Inc. Available at: https://www.endress.com/en. (Accessed: 21st August 2020)
- High Performance Kaiser bIO-Optic | Kaiser Optical Systems, Inc. Available at: https://www.endress.com/en. (Accessed: 21st August 2020)
About Kaiser Optical Systems, Inc.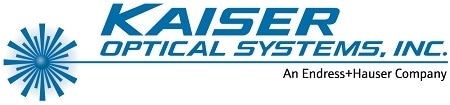
Kaiser Optical Systems, Inc. (Kaiser), an Endress+Hauser company, is the global leader in Raman spectroscopic instrumentation for laboratory, process, and manufacturing environments. Our solutions harness the powerful analytical information of Raman Spectroscopy to help our customers understand, measure, and control their chemistries.
As a trusted partner in Raman for over 30 years, Kaiser has a long history in production, including GMP manufacturing, with many proven successes. Our unmatched expertise, high quality solutions, and exceptional customer service sets Kaiser far above any other Raman option in the marketplace. Kaiser Raman technology is currently used throughout the chemical, food and beverage, oil and gas, pharmaceutical, and biopharmaceutical industries to optimize process efficiency and deliver quality products. Kaiser’s manufacturing and headquarters facility is in Ann Arbor, Michigan.
Sponsored Content Policy: News-Medical.net publishes articles and related content that may be derived from sources where we have existing commercial relationships, provided such content adds value to the core editorial ethos of News-Medical.Net which is to educate and inform site visitors interested in medical research, science, medical devices and treatments.