Dr. Peter Sobolewski and his colleagues at the Department of Polymer and Biomaterials Science at the West Pomeranian University of Technology, Szczecin (ZUT), is leveraging the power and ease-of-use of the Henniker HPT-100 benchtop plasma system in their work.
The instrument provides valuable insight into the team’s work in polymeric biomaterials.
Biomaterials are engineered materials that have been designed to interact with biological systems, for example, when implementing a medical implant or other devices.
Increased interest in the regenerative medicine and tissue engineering fields has driven the development of new biomaterials, most notably polymers destined for use as scaffolds for tissue regeneration or in soft tissue implants.
Dr. Sobolewski and his colleagues are focused on synthesizing and studying these polymeric biomaterials.
The end-use forms of biomaterials are highly varied, ranging from nanoparticles to nanofibers and microfibers or macroscopic objects such as implants, sutures or bandages. Despite this variety, biomaterials’ surfaces play a critical role in their ‘biocompatibility.’
In this context, biocompatibility represents the material’s ability to perform its designated function while being tolerated by the host’s tissue, therefore producing an appropriate host response.
A diverse array of techniques have been employed in the study of biomaterials’ surfaces, as well as their interactions with cells and proteins.
One means of studying polymer surface interactions and properties involves the careful preparation of precisely controlled thin films via spin coating.
Depending on the study in question, a substrate, such as a glass coverslip or sensor, is coated with a layer of polymer in the range of 100 to 300 nm. This is done by depositing the polymer in solution and rapidly rotating the substrate to ensure the film spreads evenly and the solvent evaporates.
The films must be pristine and free of defects (for example, pinholes) or contaminants (for example, dust) if reliable data is to be obtained. If the substrate is not pristine, the film will not be pristine. Cleaning and preparing substrates for the spin coating procedure is, therefore, critical.
Dr. Sobolewski and his colleagues are currently looking into a family of poly(butylene succinate-dilinoleic succinate) co-polyesters (PBS-DLS) that were recently developed in their department.
These copolymers exhibit excellent biodegradability and tuneable elastomeric properties. They can also be synthesized from bio-based monomers through the use of non-toxic catalysts, including enzymes.
Dr. Sobolewski recently investigated the interactions between these polymers and two proteins that play a central role in cell-material and wound healing interactions: fibronectin and fibrinogen. These proteins are both components of the provisional matrix.
The study saw spin-coated thin films being prepared on quartz crystal sensors before being analyzed using a quartz crystal microbalance (QCM).
The results suggested the potential for excellent cell-polymer interactions, but direct visualization of cell adhesion was difficult because the quartz substrates are not transparent.
Master’s student Nina Kantor-Malujdy also faced this challenge when working to prepare analogous thin films on a transparent substrate. She worked with Dr. Sobolewski to select glass coverslips as appropriate substrates before optimizing the spin coating process to achieve robust results.
The team realized that the as-manufactured glass coverslips had been contaminated with organic residues, making it difficult to ensure pristine coatings.
Nina Kantor-Malujdy addressed this by following an already-established cleaning protocol, utilizing sonication in isopropyl alcohol then acetone, before finally drying this using filtered compressed air.
While it was effective, this protocol was also time-consuming, low-throughput and required a large amount of manual handling and manipulation of each coverslip - especially during drying.
ZUT case study: Plasma cleaning biomaterials
The team opted to utilize plasma cleaning using their new Henniker HPT-100 instrument, having obtained the protocol from an article tweeted by Henniker Plasma.
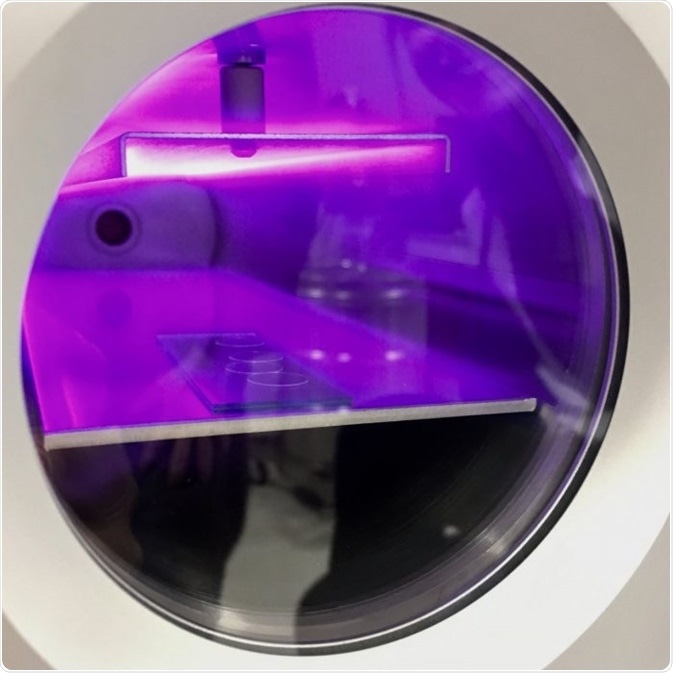
Figure 1. Plasma cleaning coverslips using HPT-100. Master’s student Nina Kantor-Malujdy seen in the reflection. Image Credit:
The specific process parameters that the team employed were: 30 seconds at 100% power, with 50 sccm airflow. It was noted that the effects were instantly apparent, with the water contact angle reducing from ~80° to virtually zero, versus ~60° for the solvent cleaning method.
Not only were the organic contaminants removed, but it was also observed that the glass became activated, resulting in it exhibiting a high surface free energy.
Nina Kantor-Malujdy verified that the effect could be leveraged in the next steps of her process. She monitored the water contact angle over time, noting that this was still ~5° after 24 hours. This left ample time for the spin coating stage of the process.
Using laser scanning microscopy, it was possible to confirm the glass was free of any contaminates but that this had not been etched. This was evident because the roughness parameter (Ra) was reduced from 11 nm as-manufactured to 10 nm - both measurements were almost at the sensitivity limit of the technique.
Using the Henniker plasma system significantly reduced the time of preparing samples because cleaning the cover glasses was an integral part of the process and previously was laborious and took a substantial amount of time.
Plasma cleaning yielded clean and dry coverslips in 30 seconds, without etching the glass or unnecessary handling. The Henniker instrument was easy to operate with a user-friendly interface.”
Nina Kantor-Malujdy
With the substrate preparation performing as required, it was possible to focus on the spin coating process. The protocol developed for quartz crystal sensors was not viable for glass coverslips, so it was necessary to develop an optimized spin coating protocol.
The team discovered that the time saved on cleaning the glass coverslips proved essential, and it was also possible to change solvents from dichloromethane to the less volatile tetrahydrofuran.
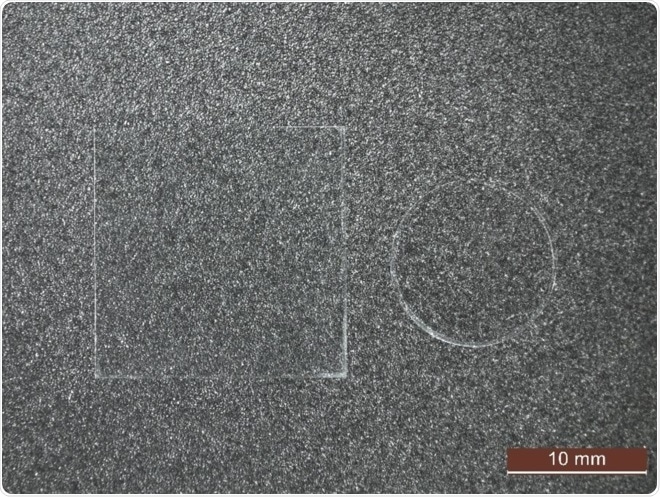
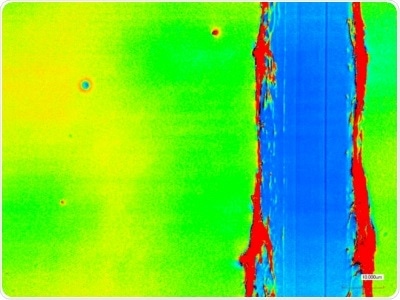
Figure 2. Top: Two types of coverslips spin-coated with PBS-DLS 50:50 copolyester (1.5 wt.% solution in THF, 4000 rpm) Bottom: Laser scanning micrograph of a scratch test of the coating on round coverslip confirming ~100 nm thickness and Ra ~10 nm.
It was also possible to reliably acquire PBS-DLS copolymer coatings ~100 nm thick with similar roughness to the substrate (Ra ~10 nm). These coatings replicated the polymer surfaces employed in the QCM protein adsorption studies.
With these factors and optimizations in place, the team was ready to begin the planned cell adhesion studies.
Before the lab had to be shut down due to the COVID-19 pandemic, the team performed an initial experiment using L929 mouse fibroblasts - a workhorse cell line commonly used in preliminary biomedical studies.
This experiment offered very promising results, and it was noted that after 24 hours in culture, there was robust adhesion of cells to the experimental copolymers. It was also observed that the cells had a similar morphology to tissue culture plastic control surfaces.
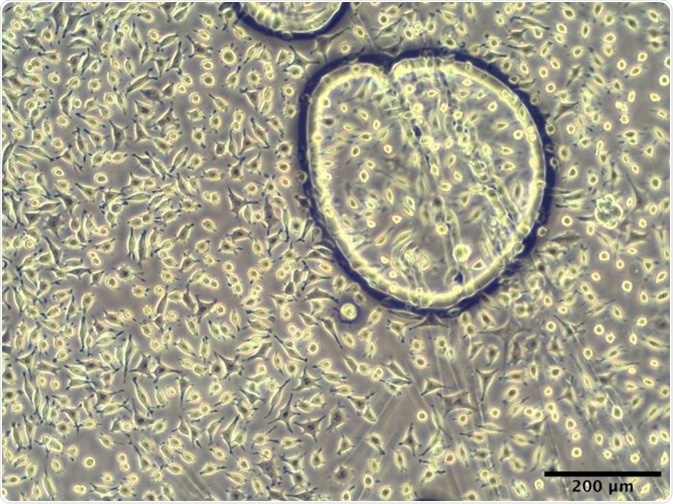
Figure 3. L929 murine fibroblasts adhered to PBS-DLS 50:50 copolyester spin-coated surface after 24 hours of culture.
Now that the COVID-19 pandemic has subsided enough to allow the team to return to the lab, they plan on undertaking careful quantification and replication of the initial study. They also expect to begin working with live-cell imaging in order to monitor adhesion in real-time.
It can be challenging to keep cells alive and healthy during experimental manipulations and live-cell imaging. It is, therefore, essential that the methodology for preparing the substrates is as reliable and reproducible as possible.
The use of the Henniker HPT-100 plasma system and the diligent work of Nina Kantor-Malujdy enabled these aspects to be optimized and streamlined for the study in question.
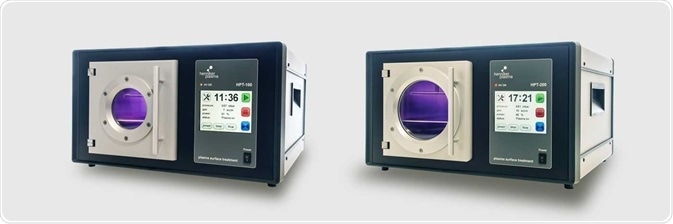
Figure 4. The Henniker HPT-100 & HPT-200 Plasma Systems.
“These coatings are a model of the surface of a device that may be an implant in the body. To determine the biological properties and thus optimal applications of these new co-polyesters, we need to study whether cells will attach and proliferate on such surfaces,” Nina Kantor-Malujdy elaborates.
The complexity and variability of biological systems make our studies challenging, so I was extremely happy that the process of cleaning and spin-coating the glass coverslips could become simple and routine. I’m grateful for the excellent work of Master’s student Nina Kantor-Malujdy.
Regarding the plasma system, we needed a cost-effective plasma system that could be easily set up and produce the right process for us. We were already thinking about cleaning samples but also wanted to use it for activating polymer surfaces. Finally, we’d like to move into the area of plasma polymerization.
We chose the HPT-100 plasma system from Henniker Plasma, because of the flexibility of the system and the excellent support from Henniker Plasma and Polish representative Jacek Łatkowski of EDFelectronics.”
Dr. Sobolewski
References
- Stepień, K.; Miles, C.; McClain, A.; Wiśniewska, E.; Sobolewski, P.; Kohn, J.; Puskas, J.; Wagner, H. D.; el Fray, M. Biocopolyesters of Poly(Butylene Succinate) Containing Long-Chain Biobased Glycol Synthesized with Heterogeneous Titanium Dioxide Catalyst. ACS Sustainable Chemistry & Engineering 2019, 7 (12), 10623–10632. doi.org/10.1021/acssuschemeng.9b01191.
- Sonseca, A.; el Fray, M. Enzymatic Synthesis of an Electrospinnable Poly(Butylene Succinate-Co-Dilinoleic Succinate) Thermoplastic Elastomer. RSC Adv. 2017, 7 (34), 21258–21267. doi.org/10.1039/C7RA02509B.
- Sobolewski, P.; Murthy, N. S.; Kohn, J.; el Fray, M. Adsorption of Fibrinogen and Fibronectin on Elastomeric Poly(Butylene Succinate) Copolyesters. Langmuir 2019, 35 (26), 8850–8859. doi.org/10.1021/acs.langmuir.9b01119.
About Henniker Plasma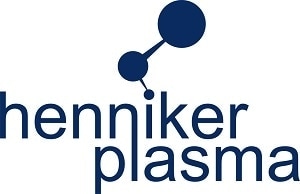
Henniker – Passionate about Plasma®
We are an experienced, dynamic and expanding company already established as a leading UK manufacturer of plasma treatment systems for cleaning, activation and coating.
Our success is built around an exceptional body of knowledge and expertise, backed by highly trained and dedicated staff who understand your application in considerable detail. Our standard configurations cover most applications but we understand too that no two samples or surfaces are the same. That’s why, uniquely, we offer a wide range of options that allow us to customise any standard system for your exact requirement.
Sponsored Content Policy: News-Medical.net publishes articles and related content that may be derived from sources where we have existing commercial relationships, provided such content adds value to the core editorial ethos of News-Medical.Net which is to educate and inform site visitors interested in medical research, science, medical devices and treatments.