Please can you give an introduction to your research?
I'm a professor in the Department of Integrative Structural and Computational Biology at The Scripps Research Institute. I have been performing NMR research on proteins for nearly 40 years.
In very general terms, my research is focused on the relationship between the structure, dynamics, interactions and function of proteins. In some projects, we focus purely on dynamics, trying to understand how the dynamics of enzymes, for example, relates to enzyme catalysis and interactions. We are also interested in how transient fluctuations in a protein structure can lead to aggregation-prone states that cause disease.
A major research effort in the lab is focused in the area of intrinsically disordered proteins or IDPs. This is a large part of our research activity and has been for very many years, ever since we stumbled across IDPs in the mid-1990s. An enormous effort is being made in the lab to try to understand IDPs, their conformational ensembles, and their biological functions.
The Dark Proteome - NMR in Disease Research
The Dark Proteome - NMR in Disease Research from AZoNetwork on Vimeo.
How important is it to study IDPs?
It is extremely important. IDPs represent about one third of the proteins in the human proteome. We know very little about them and refer to them as the “dark proteome”.
IDPs are involved in a huge number of functions in the cell. They control cellular regulation and signaling and are involved in organization of membrane-less compartments in the cell. They are closely associated with disease and are linked to cancer, diabetes, cardiovascular disease, infectious disease and neurodegenerative diseases such as Alzheimer's and Parkinson's disease.
IDPs are incredibly important in human biology and, obviously, in medicine. IDPs offer us new possibilities to develop novel drugs and identify novel therapeutic targets, so it is extremely important to study them and understand how they function.
Why is this area so challenging?
It is challenging for very many reasons. They pose many challenges for NMR, which is the central tool for studying IDPs. Traditional structural biology methods such as crystallography cannot be used for IDPs because they do not have unique structures.
When IDPs are in the free state and not bound to targets, they adopt ensembles of structures, but they frequently also form dynamically disordered complexes when they interact with a target.
The method of choice for studying IDPs is NMR, but they pose real challenges because the dispersion in the NMR spectrum is very limited. If an IDP is highly dynamic, then the lines are very sharp and you can apply multidimensional, 4D and 5D experiments to resolve the poorly dispersed spectra and minimize peak overlap.
However many IDPs actually have broad cross peaks due to local compaction of the IDP and restricted motions. For large IDPs, the spectra frequently contain a mixture of sharp and broad resonances and that poses additional challenges.
Another major challenge is that there is frequently exchange broadening in IDP complexes due to the timescale of exchange between free and bound states or fluctuations within the complex that cause broadening of the NMR signals. This is another major challenge that needs to be addressed in NMR studies of IDPs.
A final challenge is that many eukaryotic proteins contain both globular domains that are well structured and disordered regions. One needs to understand these proteins at a holistic level: how do the ordered and disordered regions function together to perform the biological function? Again, this poses a real challenge because you typically get broad signals from the globular regions and sharp signals from the disordered parts.
Can you share with us some recent results of your research?
Firstly, NMR is a central and arguably the most important tool for studying disordered proteins. One of the reasons for this, is that NMR can be used to characterize the conformational ensemble of an IDP, follow post translational modifications, and characterize the protein when it is in the free state as well as when it is bound to its target.
The first example I will give is the work of two Japanese post-docs, Kenji Sugase and Munehito Arai, who have used NMR relaxation dispersion experiments to characterize the mechanisms by which disordered proteins bind to their targets. They were able to show that the mechanism of binding and folding depends on the population of folded states in the conformational ensemble of the free IDP.
If there is a high population of native-like folded states in the conformational ensemble, say more than 40 or 50%, then this dictates one folding pathway - the protein folds before it binds. If the conformational ensemble of the unbound state is deficient in natively folded states, then folding occurs after binding.
Relaxation dispersion experiments provide insights into the kinetics and mechanism of the IDP binding processes. This is very important information to guide the future development of novel drugs.
The second example I will give deals with a problem that is encountered quite frequently in doing NMR spectroscopy on complexes of IDPs to gain understanding of how they interact with their targets. One of the very real challenges is that when complexes are formed, the NMR spectrum is often exchange broadened, making it very difficult to assign resonances and determine the structure of the complex.
A way around that problem was developed by a graduate student in the lab, Alexander Krois, and a post-doctoral fellow, Josephine Ferreon. By fusing the IDP to its binding target in a single polypeptide chain, the cross peaks in the NMR spectra became sharper and they were able to make complete assignments and determine the structure.
Using this strategy they were able to obtain structures of the full-length activation domain of the tumor suppressor p53 bound to a target protein, leading to new understanding of the intermolecular interactions.
However, one of the downsides of this strategy is that proteins must be isotope labeled for NMR experiments; if a fusion protein is labeled with isotopes, then both the disordered protein and its target become labeled. As a consequence, the NMR spectra of the fusion protein become a lot more crowded and a lot more complicated than the spectra of the individual components.
Alex came up with a very neat solution to this problem by using inteins. By fusing the two proteins using inteins, he could label the IDP with N15 and C13, while leaving the target protein unlabeled, or vice versa. This resulted in simplification of the spectra and facilitated structure determination and studies of dynamics.
You can also take the intein strategy a step further. The method Alex developed is very robust and can be applied to proteins that contain a combination of disordered regions and globular regions. Using inteins, the globular region can be left unlabeled while just the disordered part is isotope labeled. Alex demonstrated this strategy using the tumor suppressor p53.
By making the resulting segmentally labeled constructs, he can then use NMR to probe interactions within the full length p53 tetramer. He obtains beautiful spectra of the disordered region, and can follow post translational modifications and interactions with other molecules without the complexity of the broad signals coming from the folded core domain of p53.
How important is NMR? What does it give you that other technologies do not?
NMR is a central tool for the study of IDPs. It provides technology to characterize the conformational ensemble of IDPs, leading to new understanding of the conformations that are populated in in the free state and the dynamics with which they exchange.
NMR also allows characterization of IDP complexes, which are frequently partly disordered. There are two extremes; sometimes when an IDP binds to its target it will fold up into a well-defined structure; other IDPs remain dynamically disordered in their complexes with their binding partners.
In some cases, an IDP is partly structured and partly disordered when bound to a target protein. This gives rise to the concept of static and dynamic binding sites, where part of the protein folds on binding while other parts remain dynamic in the bound state. NMR is the only tool that can be used to investigate these complex and dynamic interactions.
The ability to characterize dynamic proteins, to map conformational ensembles, to follow post translational modifications in real time, and to determine the structure and dynamics of IDP complexes is what makes NMR such a powerful tool for studying IDPs. NMR is also a very powerful tool for studying interactions, since it is straightforward to identify the regions of an IDP that interact with its target through changes in chemical shift or line width.
How will your work impact medicine and biomedical research?
It will do so in many ways. Obviously this is a major challenge, since one third of the proteins in the human proteome are disordered, and the range of their functions and behavior is vast. New and totally unexpected results are constantly emerging in the IDP field. The concept that disordered proteins undergo phase transitions and form membrane-less compartments within the cell, for example, was totally unexpected. An enormous amount of work needs to be done to understand IDPs and their function in the cell.
Our own work is beginning to provide new insights into the mechanisms by which disordered proteins bind to their targets, the molecular basis by which they recognize their targets and how they regulate signaling pathways. Much of the complexity of the signaling pathways in a cell comes from disordered proteins and the post translational modifications that they undergo.
Our research is providing necessary technologies for study of IDPs and we are beginning to scratch the surface of understanding how IDPs are functioning in the cell. As we learn more about how disordered proteins function and what their role is in signaling and disease, new possibilities open up for the design of therapeutics.
Part of our research that impinges directly on this concerns viral IDPs. Many viruses express intrinsically disordered proteins in an infected cell that function to hijack the cellular regulatory networks. Many of the DNA tumor viruses, for example, produce disordered proteins that block the action of p53.
The tumor viruses hijack the host cell cycle by using their own disordered protein regions to mimic cellular IDPs. We should be able to do the same thing with drugs, by designing small molecules or peptide-like molecules that target the cellular machinery in the same way that viral IDPs do. These are the types of insights that we gain from studying disordered viral proteins and their interactions that will have an enormous impact in the long term.
Which specific proteins are you focusing on and how do they relate to disease?
Many of the proteins that we are studying are directly related to disease. One such disease is cancer, which we are addressing through our work on the tumor suppressor p53 and DNA tumor virus proteins. We are trying to understand the molecular mechanisms by which DNA tumor virus IDPs function to hijack cellular signaling pathways and disrupt regulation of the cell. These projects relate directly to disease.
%20is%20a%20transcription%20factor%20whose%20inactivation%20can%20trigger%20the%20onset%20of%20cancer-petarg.jpg)
Another area that is disease related is directed towards understanding how a protein can transiently unfold, either partly or fully, to form an aggregation-prone state that causes disease. We are focusing on the protein transthyretin, which is not an IDP.
Many pathogenic mutations occur in transthyretin and these mutations lead to early onset amyloid disease if you are unfortunate enough to have one of those mutations. However, about 25% of octogenarians suffer from senile systemic amyloidosis, a cardiac disease in which wild type transthyretin unfolds and aggregates.
Given the prevalence of this disease in the elderly population, understanding how the wild type protein unfolds to form amyloid is extremely important. Again, we use NMR relaxation dispersion methods to observe a transiently populated aggregation-prone state, populated to the level of only 1- 2%.
There is so little of this state formed that it can’t undergo aggregation- it simply relaxes back to the correctly folded ground state. However, by using NMR relaxation dispersion we can characterize the structure of the transiently unfolded state that leads to aggregation and disease.
How will your work impact the future of biomedical research?
I think the impact will be very high. The IDP field is huge. As I mentioned before, about one third of proteins in the human proteome are disordered. It's going to take an enormous effort to understand the role of these proteins and the impact of the dark proteome upon cellular signaling and the organization of cells, as well as its impact upon medicine.
The work that we are doing is laying some of the foundations for a mechanistic understanding of how IDPs function - how they function in signaling and how they interact with their binding partners. Understanding of these processes is going to be very important in the future for developing new drugs and therapeutics to target diseases associated with IDPs.
.%20A%20model%20is%20built%20using%20data%20of%20viral%20macromolecular%20structure%20from%20Protein%20Data%20Bank%20(PDB%203J6R)-Kateryna%20Kon.jpg)
One of the problems we are studying in the lab concerns the mechanism by which disordered virus proteins hijack the machinery of the infected cell. We are particularly interested in DNA tumor viruses such as the human papillomavirus, which causes cervical cancer, and are trying to understand how the disordered proteins produced by HPV and other DNA tumor viruses interact with cellular targets, competing with cellular IDPs to hijack the cellular machinery.
Viruses are very effective drug designers. Over billions of years, they have evolved to target the cellular machinery in ways that we cannot. I think that there is a real lesson for us there. An understanding of how viral IDPs function in the cell can guide us to design our own molecules to disrupt cellular pathways and treat disease, both viral disease and other diseases. We can use the viral IDPs as a model to gain new insights into how to target cellular pathways and control cellular regulation.
About Peter Wright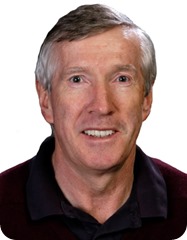
Peter Wright is a Professor in the Department of Integrative Structural and Computational Biology and holds the Cecil H. and Ida M. Green Chair of Biomedical Research at The Scripps Research Institute.
He received his B.Sc., M.Sc. and Ph.D. degrees from the University of Auckland, New Zealand, and undertook postdoctoral studies at Oxford University, UK, from 1972–1976. He joined the faculty at the University of Sydney, Australia in 1976. He was appointed to the faculty of Scripps in 1984 as Professor, and was Chairman of the Department of Molecular Biology from 1987-2012.
His research has focused on applications of nuclear magnetic resonance to study mechanisms of protein folding, the structural basis of protein-protein and protein-nucleic acid interactions in the regulation of gene expression, and the role of dynamics in protein function.
His work on protein interactions led to the realization that many proteins do not adopt stably-folded, globular structures but are intrinsically disordered, and that protein disorder plays an important functional role in cellular signaling networks.
He is an elected fellow of the International Society of Magnetic Resonance, the NMR Society of Japan, the American Association for the Advancement of Science, and the American Academy of Arts and Sciences, and is a member of the National Academy of Sciences. He is Editor-in-Chief of the Journal of Molecular Biology.
About Bruker
Bruker is market leader in analytical magnetic resonance instruments including NMR, EPR and preclinical magnetic resonance imaging (MRI). Bruker's product portfolio in the field of magnetic resonance includes NMR, preclinical MRI ,EPR and Time-Domain (TD) NMR. In addition.
Bruker delivers the world's most comprehensive range of research tools enabling life science, materials science, analytical chemistry, process control and clinical research. Bruker is also the leading superconductor magnet and ultra high field magnet manufacturer for NMR and MRI solutions.