Sponsored Content by PittconApr 24 2020
In this interview, Professor John Rogers talks to News-Medical Life Sciences about his research and work in developing biocompatible electronics and microfluidic systems with skin-like properties.
Biological systems are traditionally mechanically soft however modern electronic and microfluidic technologies are rigid, meaning the layouts are completely different. Eliminating this mismatch will create huge opportunities in man-made systems that can be used for diagnostics, therapeutics and in clinical and healthcare. Can you tell us about the new opportunities these man-made systems will create?
There are all kinds of interesting and compelling opportunities that could come from thinking about how to reformulate the kinds of systems that form the core foundations of devices that you see in consumer gadgetry, so computer chips, integrated circuit chips that are flat and rigid and planar, into forms that are more naturally biocompatible – compatible with the soft surfaces of the human body. And, integrated circuits are not the only kind of man-made technology that has those kinds of physical characteristics and geometrical shapes.
You see the same type of thing in optoelectronic devices, lab-on-a-chip type technologies and microelectromechanical systems. The goal behind our research, and that of a growing community of researchers, is to create new ideas in material science and manufacturing, mechanical engineering and electrical engineering that will allow us to reformulate those sorts of technologies, without sacrificing the performance or capabilities, into platforms with geometries and mechanical properties that are inherently biocompatible and can be interfaced with soft tissue systems -- the skin, the brain, the heart, the peripheral nervous system, the bladder, and the kidneys. And the idea is to develop those technologies into system that can ultimately enhance human health and extend life.
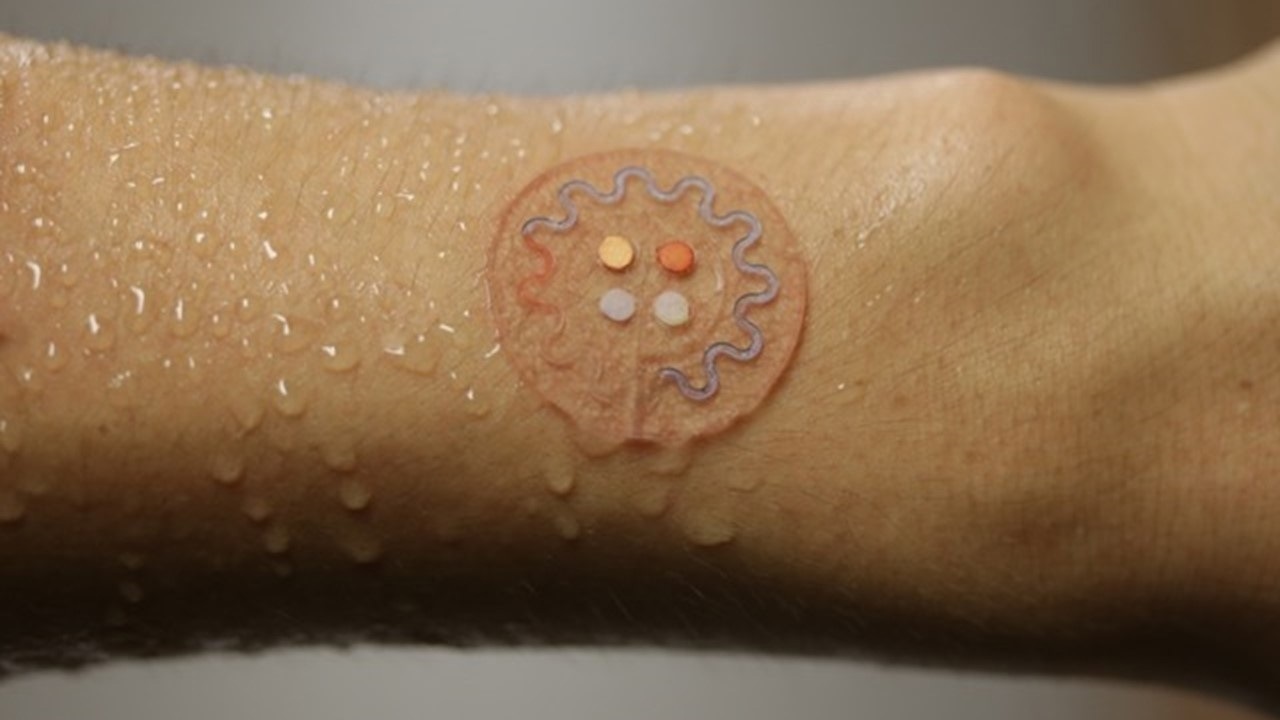
Image Credit:Rogers Research Group - NorthwesternUniversity
Can you tell us more about these ‘biocompatible’ electronic and microfluidic systems with skin-like physical properties?
The skin-like devices that we have developed are specifically designed to interface with the skin and to use the skin as a window for measuring clinical-grade physiological status parameters associated natural processes of the human body. For example, looking at cardiac activity, respiratory activity, flow properties associated with blood through near-surface arteries and veins; to reproduce what's done in the hospital, but in platforms that can be worn continuously for wireless streaming of data outside of the hospital in the home setting, to develop a deep foundational basis of information on health status. This information can be used with artificial intelligence algorithms to assess a person's well-being at any given moment and to make predictive assessments of health trajectories over time. This kind of personalized, digitally oriented model for healthcare enabled by these kinds of skin-like platforms will be a very powerful way that healthcare will evolve into the future for reduced costs and improved outcomes.
You presented the Wallace. H. Coulter Lecture this year at Pittcon 2020 in Chicago. What did you discuss in your talk?
In this talk, I will focus on skin-interfaced systems, devices that provide not only this electronic monitoring functionality but those that also embed tiny networks of microchannels. The microchannels along with very small reservoirs and valves that are designed to capture sweat that is pumped to the surface of the skin through the Eccrine glands and the connective ducts for capture and analysis of biomarkers in sweat.
Sweat is a relatively under-explored but very potentially important class of bio-fluid that could provide information content to substitute for blood draws. The idea is to use sweat and the noninvasive ability to collect sweat to do biochemical based assessments of health status to complement the sort of physics-based measurements that we can achieve with our electronic devices.
The Analysis of Sweat in Performance and Health Management from AZoNetwork on Vimeo.
What sparked your interest in ‘soft’ materials?
My core expertise is in electronic materials, and I like to think about novel electronic materials in the context of technologies with capabilities that go beyond what is currently supported with conventional sorts of electronic materials. We got our start in this area thinking about flexible displays, so paper-like displays that could replace the kind of liquid crystal and organic light-emitting diode displays that you see in consumer devices today. So, thinking about thin paper-like systems, lightweight, mechanically rugged, capable of rolling up in storage when they're not being used. And so that was interesting for us for a while, and it remains a major focus at most large display companies.
I happened to be giving a talk at the University of Pennsylvania on that kind of technology. It turned out that a couple of curious neuroscientists were in the audience and they came up to me after the talk and asked whether we could take those kinds of flexible electronic devices and put them on the brain to study the electrical activity of the brain. That was the first suggestion that these kinds of devices could be brought to bear to important problems in human health and in research around the fundamental mechanisms that govern the behavior of living systems. That interaction catalyzed a whole new set of research opportunities for us and it has been a sustained area of activity now in the group for the last 10 years.
What extra levels of functionality do soft electronics provide? How were they discovered?
Soft electronics allow you to intimately and persistently integrate advanced biosensors, radios, stimulators, microprocessors and digital memory technologies with the human body, in ways that go far beyond what's possible with conventional wearable technologies that you see on the market today. Commercial devices are dominated by bulky, clunky pieces of electronics, loosely strapped to the body, typically at the wrist. That kind of technology approach can allow you to measure certain parameters, qualitative assessments of health and wellbeing, you can count steps, you can get a rough estimate of heart rate, but those are parameters that physicians can't readily interpret and act upon.
What we're thinking about is the next generation of wearable technology that integrates more intimately with the body, almost serving as a second skin that laminates in a physically imperceptible way on the surface of your actual skin. Almost like a temporary tattoo or a bandaid to provide ICU grade measurements of health status to allow physicians to, at a very detailed level, track health progression over time, not in an episodic way, which is currently the way that measurements are made when a patient comes to a hospital or a clinic. But now thinking about those same types of measurements performed continuously and I feel that it's going to open up new frontiers to think about how to manage health conditions and to promote healthy living as well.
What ‘skin-like’ physical properties do biocompatible electronic and microfluidic systems have?
We target a set of physical properties that are precisely matched to the skin itself. The skin stretches somewhat, it can flex and bend and wrinkle, it has certain thermal characteristics, it has a whole set of properties, water permeability characteristics as well, thermal. And we try to embody those exact skin-like properties into electronic devices. And that's the trick and the centroid of the research that we've been doing over the last decade or so. You think about a silicon-based integrated circuit, the mechanical properties are a million times different than those of the skin. So, there's a huge chasm and a gap there. It's perfectly flat, it really can't conform to a natural curvature and the sort of sub-millimeter scale texture associated with the skin. So we try, to the best of our ability, to take a collection of materials and build them into an electronic system that has the same type of functionality you achieve with a silicon-based electronic platform today, but with mechanical properties, geometrical features that precisely match those of the skin.
The goal is to develop almost like a second skin that interfaces directly and naturally with your natural skin so you can wear these devices for long periods of time without even realizing they're there because they're matched to the skin. You don't have a physical sensation that they're there. And we think that that's not just a convenience, that's an essential characteristic of the devices because if they can't be worn in a comfortable way that doesn't introduce irritation at the surface of the skin, then nobody will wear the devices. The patient compliance will be unacceptably low.
That's the goal around engineering and it turns out that with a few relatively simple ideas, we can get very close. The thickness of these devices is thinner than the epidermis, the mechanical properties almost precisely matched, the overall thermal mass is almost the same, there's no thermal load as a result.
Could you name some of the main advantages of using soft, skin-like electronics over using conventional hospital apparatus?
What's used in hospitals today, are primarily biosensors that are attached to the surface of the skin just with adhesive tapes. They connect via hard wires to external boxes of electronics that do all the data acquisition and storage and processing. And that works fairly well for an adult patient who's in a hospital bed and not moving around a lot.
The wires, however, even in that kind of scenario, create a pretty serious inconvenience and in many cases, they frustrate basic operations in clinical care, surgical operations are confounded by the presence of the wires.
The idea is to go to a platform that gets rid of the wires, so it's wireless. And the forces that are inevitably imparted through the wires to the interface between the biosensors and the skin also go away, we can get away with an adhesive that has an adhesive strength to the skin that's a factor of 10 or a hundred times lower than that which is required for the wired based devices. And the consequence of that is that you end up with a much more comfortable interface to the skin and one that is much less prone to create skin irritation.
In the context of almost all sorts of hospital practice in terms of monitoring, these kinds of skin-like or band-aid-like devices represent an important advantage, but if you take a look at probably the most extreme scenario where those wires are problematic, it's what you encounter in the neonatal intensive care unit.
If you consider what's done with premature babies, they have to be monitored for all vital signs, at clinical grade quality 24/7, because they're in a very fragile health status, but their skin is also very fragile. They don't accommodate these strong adhesive tapes very effectively. The wires are not just a nuisance, now they frustrate the natural motions of the baby. They frustrate the ability of the parents to interact with the baby as well because you have to manage the wires.
A lot of the work that we've done so far is focused on that use case as the most compelling opportunity for these kinds of technologies. We've done a lot of work in the NICU facility at Lurie Children's Hospital in Chicago. We tested out the devices on about a hundred neonates who've come through the hospital and we’ve shown equivalency in the measurements made with our wireless skin-like devices to those determined with the conventional wired based devices and external boxes of electronics.
In fact, those platforms are now deployed at scale in Africa through funding from the Gates Foundation and the Save The Children Foundation, because in the developing world there are no monitoring capabilities at all. The idea is to kind of leapfrog the old-style wired based devices, go straight to wireless and provide improved capabilities in healthcare in that context of neonatal, pediatric, maternal and fetal health.
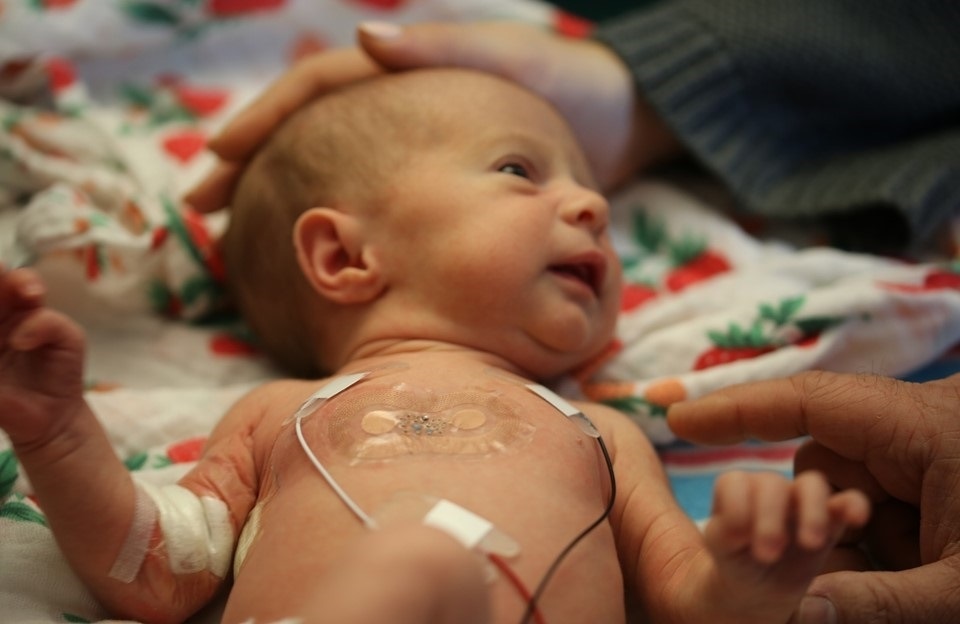
Image Credit:Rogers Research Group - NorthwesternUniversity
Another application of biocompatible electronic systems is in sport and fitness research. Why is sweat an important bodily fluid to be looked at?
Sweat, in the context of athletics, athletic performance, fitness, and general well-being is sort of low hanging fruit in terms of how to think about sweat as a biofluid, that can characterize health because it's very clear that sweat loss can lead to dehydration.
In fact, maintaining optimal sports performance requires optimal hydration management. If you go into a training scenario or you enter an athletic competition, you want to keep your body at an optimally hydrated state. So, the ability of these skin interfaced microfluidic devices to continuously monitor sweat loss locally -- and that local measurement correlates to full body sweat loss -- can inform an athlete precisely how much water they need to drink in order to replenish the lost water through the sweating process.
But not only that, we can also measure the electrolyte concentration in sweat – a quantity that varies depending on the individual, their genetic background, their racial background, their dietary habits, all sorts of things. So the devices measure not only sweat loss but electrolyte loss as well. In this way, they allow you to replenish not only the lost water but also the lost electrolytes so you can maintain not only perfect hydration, but you can also maintain perfect electrolyte balance as well.
For competition at the highest levels, a few percentage improvements that can result from that data-driven hydration management can be very important. We have a partnership with Gatorade, and as you might imagine that kind of capability is touching on their core product, to distribute these devices to athletes, both pro athletes and youth athletes as well, to maintain better performance and also to avoid things like cramping and injuries that can also result from poor hydration management.
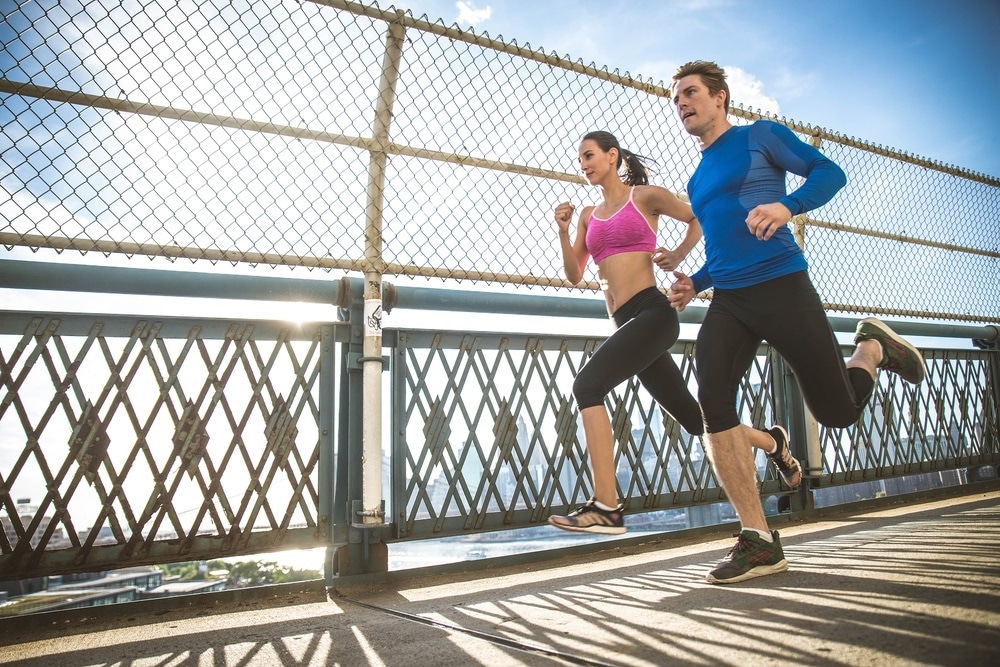
ImageCredit:Shutterstock/oneinchpunch
Nanotechnology has become an increasingly investigated area within the science industry, also having many applications within medicine. How does nanotechnology take part in your research?
Nanotechnology is important for us, but it’s not necessarily the end goal. We'll use nanotechnology where it makes sense. We're focused at the system level and how you can achieve novelty in devices and construction can yield data streams that are a direct benefit for health or fitness or sports.
But nanotechnology specifically does come into play, in a pretty simple way if you think about it. A silicon wafer has a certain set of mechanical properties that are defined by the silicon itself, but also by the geometry of the wafer. It's fairly thick, it's about a millimeter in thickness, half a millimeter in thickness or so and it's partly because of that thickness that the silicon wafer cannot be bent without fracturing the material. Nanotechnology comes into play then because reducing the thickness of the silicon imparts a flexibility to the silicon just due to elementary bending mechanics. So a 2 x 4 you can't bend that. You can bend a sheet of paper. It's the same materials just by virtue of the fact that the paper's really thin compared to the 2 x 4 that you can bend it.
The same principles apply to silicon. We deploy silicon in nano-scale forms rather than in wafer-based forms. If you take the thickness of a wafer, half a millimeter, and you shrink that down to a hundred nanometers, the flexibility improves by a factor of 1012 or something like that. It's absolutely transformative in terms of the way you think about the material. That's how nanotechnology enters the systems that we're interested in, it allows us a straightforward route to make a material like silicon flexible and skin-compatible ultimately.
Electronic Second Skin in Healthcare
Electronic Second Skin in Healthcare from AZoNetwork on Vimeo.
If this continued research is carried out into the field of biocompatible electronic and microfluidic systems, where could this take us?
The skin interfaced devices represent the most immediate opportunity because they're minimally invasive. It's very easy to get approvals for using these devices on human patients. They can easily be removed if any kind of adverse effect develops, although we haven't seen that. It's a straightforward and natural starting point for bio-integrated electronics, as the skin as an interface, at least for use in humans. We do a lot, however, with implantable systems, primarily in animal model studies as a predicate to eventually moving into use in humans.
The frontier for us is in taking the design principles that we've proven out for skin interface devices and deploying the same technology on the brain or the heart to allow similar types of functionality but in the context of internal organs. Electronically enhanced organ health is the way you can think about it. So devices that wrap the outside surface of the heart have the ability to monitor basic cardiac function, but also with the capability to deliver stimulus, therapeutic stimulus, as an advanced type of pacemaker, but one that's distributed around the outside surface of the heart. The same types of possibilities are present in the context of brain disorders as well. So I think moving these devices from the skin to internal organs in the body is a huge area of opportunity.
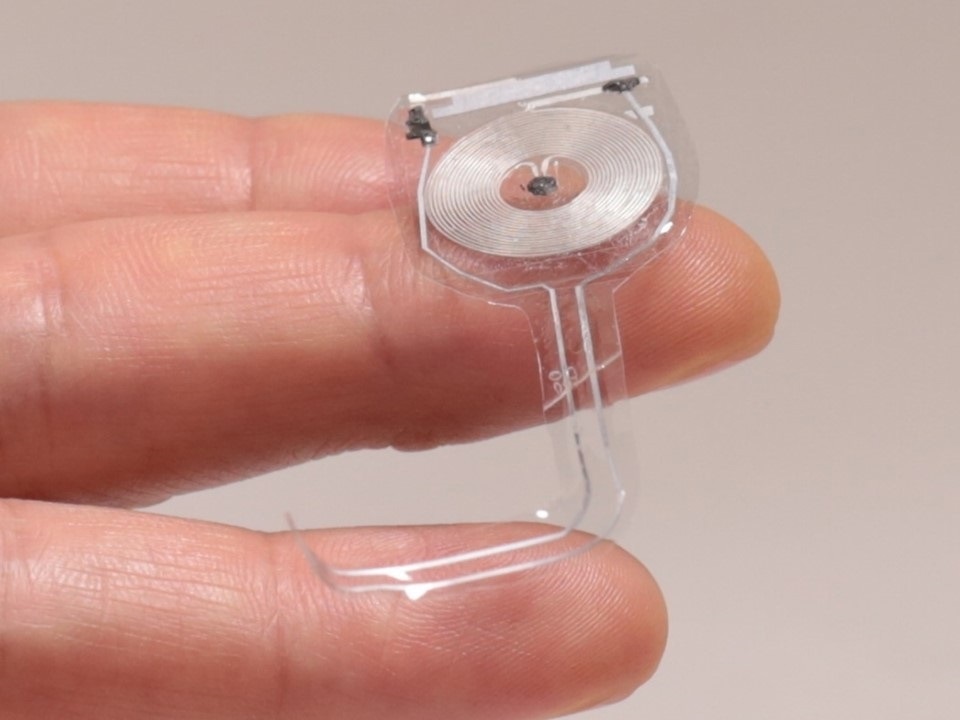
Image Credit:Rogers Research Group - NorthwesternUniversity
What is next in your research into soft, skin-like electronics?
Exploring more deeply the value and information content embedded in sweat. Sweat has not been nearly as thoroughly explored as blood or interstitial fluid as a biofluid that contains biomarkers of relevance to health status.
There's some work to be done there, but the area is opening up because now we have microfluidic devices that allow us to capture very small, but pristine quantities of sweat that can be used for very precise chemical analysis and correlation ultimately to blood.
It's a technology-enabled opportunity in studies of human physiology and basic biological questions around how sweat relates to blood. And if you can establish those correlations, then I think sweat becomes a compelling way to make a biochemical assessment of health that avoids the need to do a blood draw.
Can you tell us why you come to Pittcon?
There is an amazing collection of people who are interested in topics very similar to those that represent core activities in my own research group. There is a huge synergy and resonance between my interests and the topics that are covered at Pittcon. It's also comprehensive. It's a very large meeting with all the key experts and so it's kind of a one-stop shop for work in this area. I think it's a fantastic event and I've been to this meeting many times in the past.
What do you expect to achieve this year at Pittcon?
I'll be delivering this special lecture at Pittcon and I expect, as occurs many times, that I'll be able to strike up some conversations and seed some areas for collaboration. Conferences for me are successful if I make new connections and meet new people and maybe open up new opportunities for research.
Why are events like Pittcon important for your research but also important for the analytical chemistry industry?
The exchange of ideas is incredibly important as a catalyzing aspect of how science works. It's very important to share insights and ideas. A conference of this type provides an excellent platform for doing that, and so I think it helps everyone. It helps the whole community and helps society in a sense because it just accelerates progress in research.
About John Rogers
John A. Rogers is the Louis Simpson and Kimberly Querrey Professor of Materials Science and Engineering, Biomedical Engineering and Medicine at Northwestern University, where he is also Director of the newly endowed Center for Bio-Integrated Electronics. His research has been recognized by many awards, including a MacArthur Fellowship (2009), the Lemelson-MIT Prize (2011), and the Smithsonian Award for American Ingenuity in the Physical Sciences (2013) – and most recently the Benjamin Franklin Medal from the Franklin Institute (2019). He is a member of the National Academy of Engineering, the National Academy of Sciences, the National Academy of Medicine and the American Academy of Arts and Sciences.
About Pittcon
Pittcon® is a registered trademark of The Pittsburgh Conference on Analytical Chemistry and Applied Spectroscopy, a Pennsylvania non-profit organization. Co-sponsored by the Spectroscopy Society of Pittsburgh and the Society for Analytical Chemists of Pittsburgh, Pittcon is the premier annual conference and exposition on laboratory science.
Proceeds from Pittcon fund science education and outreach at all levels, kindergarten through adult. Pittcon donates more than a million dollars a year to provide financial and administrative support for various science outreach activities including science equipment grants, research grants, scholarships and internships for students, awards to teachers and professors, and grants to public science centers, libraries and museums.
Visit pittcon.org for more information.