Introduction
The majority of biochemical reactions occur at the phospholipid bilayer membrane, either on or within cells. These membranes affect how proteins fold as well as creating particular microenvironments where the reactions can occur. In order to emulate real biological systems, interactions should be explored in environments that mimic nature as closely as possible. This article shows how KSV NIMA’s analytical systems can be used to produce models of cell membranes and examine the biomolecular interactions that occur.
Floating Monolayer versus Supported Lipid Bilayer
In this study, Langmuir monolayers of membrane phospholipids have provided a model for studying biological membranes. Another commonly used model is the supported lipid bilayer (SLB), in which a bilayer is produced on a solid support through the Langmuir-Schaefer or Langmuir-Blodgett method. However, these methods have certain drawbacks, with unwanted interactions occurring between the support and biomolecules and lipids interfering with the diffusion of molecules. In some cases, this can cause the protein in question to denature. When the floating monolayers are used however, no such problems occur. These membrane models are also used in food technology, drug development, and biochemical and biological research.
In the following paragraphs, two case studies are described to demonstrate how these models are used. The first case study concerns the development of an anti-parasitic drug, while the second is related to methods of removing lipophilic allergenic substances from food.
Case Study I: Interaction between Anti-parasitic Peptide Drug and Cell Membrane Models
In the field of drug research, how a drug permeates the cell wall and its interactions inside the cell are important for determining its efficacy. Here, the KSV NIMA PM-IRRAS spectrometer can be employed to monitor drug candidates in vitro, prior to initiating tests in vivo. The example below uses a candidate drug for Human African Sleeping Sickness (HAT), which is caused by a protozoan parasite.
Methods
In a Langmuir monolayer model membrane of dipalmitoyl phosphorylcholine (DPCC), the interactions of an oligopeptide drug were studied. This drug, S-(2,4-dinitrophenyl)glutathione di-2-propyl ester) has demonstrated significant activity in the treatment of HAT.DPCC is a lipid commonly found in the protozoan membrane.
The drug’s monolayer properties and its interactions with the monolayers of DPPC were examined using a KSV NIMA Langmuir Trough equipped with KSV NIMA SPOT surface potential meter, as well as a KSV polarization modulation surface infrared reflection absorption spectrometer (NIMA PM-IRRAS). In addition, Brewster angle microscopy and surface elasticity analyses were performed using the system.
Results and Discussion
During Langmuir isotherm studies of the pure drug, there was an abnormal decrease in the surface pressure at molecular area of about 145 Å2 . On the other hand, no decrease was observed in the surface potential at the same molecular area, as shown in Figure 1. The potential and pressure isotherms indicate that the drug could undergo conformational change, dimerization, or aggregation at this surface pressure.
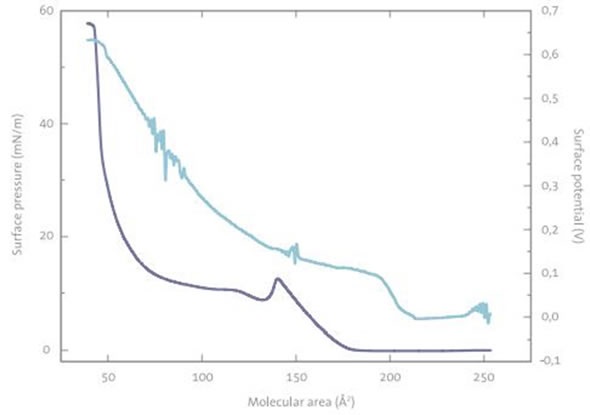
Figure 1. Surface pressure-area and surface potential-area isotherms of a HAT-drug monolayer at air-buffer interface.
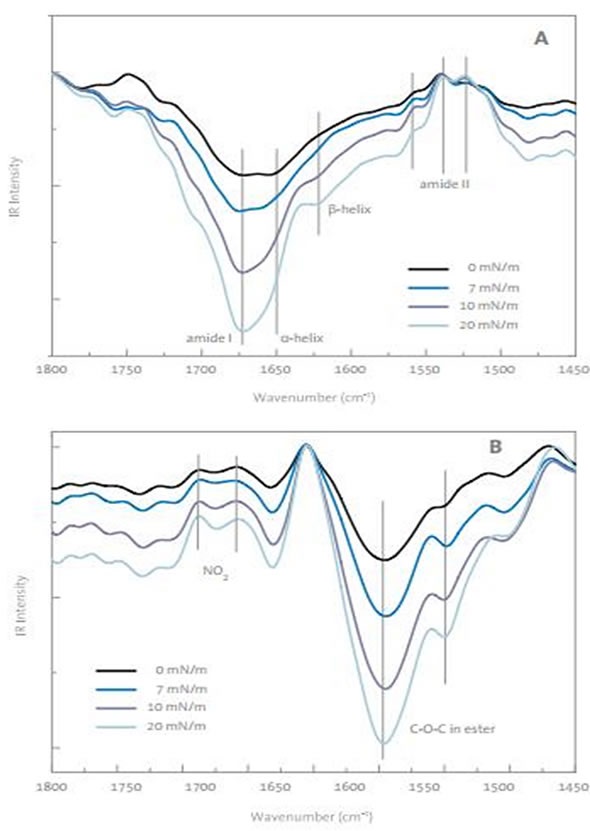
Figure 2. PM-IRRAS spectrums of drug monolayer at air buffer interface with different surface pressures. A) From 1450 to 1050cm-1 B) from 1800 to 1450cm-1.
Measurement of this monolayer by PM-IRRAS revealed negative peaks for amides, which suggested disorganized amide groups at a surface pressure of 7mN/m or less, as indicated in Figure 2A. The negative intensity for the amide suggests that the C=O groups are located parallel to the monolayer surface. Further compression of the surface caused the amide I band for beta-sheet to become more pronounced, suggesting an intermolecular relationship between the drug molecules. Given that the bands of ester and nitro-groups (Figure 2B) do not alter in their sign or position, the drug’s directional changes can be eliminated as a cause of the decline in the surface pressure isotherm. Also, the measurements of dynamic surface elasticity support the development of organized domains in the monolayer at the peak in the isotherms.
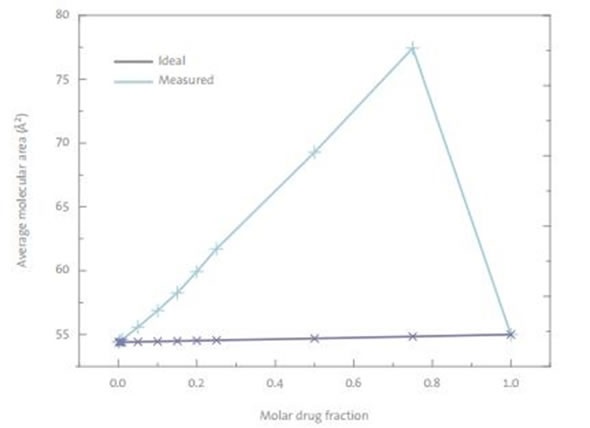
Figure 3. A plot of average molecular area of DPPC-drug mixed monolayers at a 30mN/m surface pressure. The ideal mixtures area is the arithmetic sum of the individual components at the consecutive mixing ratio.
Even small quantities of the drug were found to react with DPPC monolayers. The isotherm data (Figure 3) suggests a repulsive behavior between the drug and DPPC at all concentrations, with the relative excess area being positive for the drug. Drug concentrations as small as 0.1 mol% led to changes in the isotherm, showing that even DPPC that was not close to the drug, was affected. These phenomena are described as repulsive cooperative interactions. Even at large surface pressures of 30mN/m, the drug was not expelled from the monolayer.
The interaction of the HAT drug with the protozoan membrane model provides insight into the mechanism of the drug’s action. Even at minute concentrations, the drug affects the packing of the DPPC monolayer. The drug was also shown to associate when surface pressure was increased, suggesting the drug contained within the membrane does not get expelled at the surface pressures seen in biological systems. The interactions are also repulsive, indicating a possible disruptive action of the drug towards the membrane.
This method provides an additional screening platform for the interaction of drug candidates with the cell membrane, that may be used prior to the lengthy and costly testing of drugs in vivo.
Case Study II: Chitosan as a Removal Agent for β-Lactoglobulin
The floating monolayer model system provides a suitable method of exploring the interactions of lipophilic proteins in an environment that mimics natural cell membranes. In food processing, the removal of allergenic proteins is important in the production of health food such as milk products for allergic individuals.
In the example given below, chitosan is explored as an agent for removing β-lactoglobulin (BLG) using the KSV NIMA Langmuir Trough and the KSV NIMA PM-IRRAS spectrometer.
Methods, Results and Discussion
Using the KSV Minitrough, BLG’s adsorption behavior to the liquid-water interface was studied in both the presence and absence of lipid monolayers. This adsorption behavior was observed by tracking the development of surface pressure at constant barrier position and introducing the BLG sample to the subphase buffer. When dimyristoyl phosphatidic acid (DMPA) monolayer was present on the surface, the adsorption to the monolayer was significantly faster than when the monolayer was absent, implying an affinity of BLG towards monolayers.
In the subphase, chitosan did not promote any significant change in the surface pressure of pure buffer, but it did increase the surface pressure when introduced to the subphase in the presence of the DMPA monolayer. When BLG was injected into a chitosan-containing subphase that did not have a monolayer, a rapid increase in surface pressure occurred initially, followed by a slow increase until equilibrium was reached. The formation of chitosan–BLG complexes was also validated using dynamic light scattering measurements in bulk solution.
In the presence of the DMPA monolayer, the same experimental setup also caused a rapid increase in the surface pressure initially, which then decreased. The overall change in the surface pressure was found to be negative (Figure 4). This indicates that soluble chitosan–BLG complexes emerge at the interface. The size of the change indicates that some DMPA was also removed from the monolayer during the interaction.
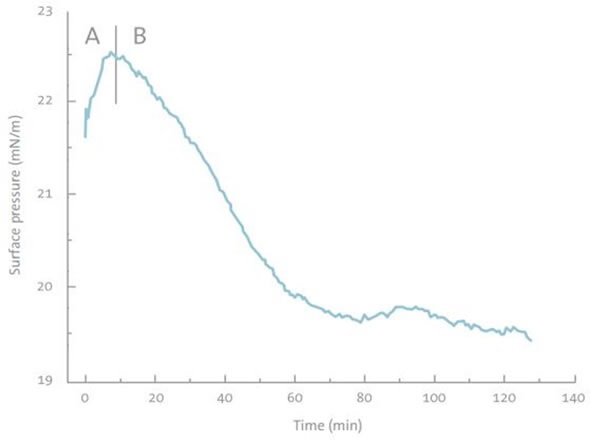
Figure 4. Absorption kinetics of BLG to the DMPA monolayer when chitosan is present in the subphase. A) BLG absorption B) BLG-chitosan complex desorption.
When the same system was tested using different monolayer lipids and a different subphase protein, chitosan could not remove BLG from a neutral phospholipid monolayer, but similar behavior to DMPA monolayer was observed for another negatively charged lipid layer. This suggests that the negative charge may play an important part in the removal of chitosan–BLG complexes from the monolayer.
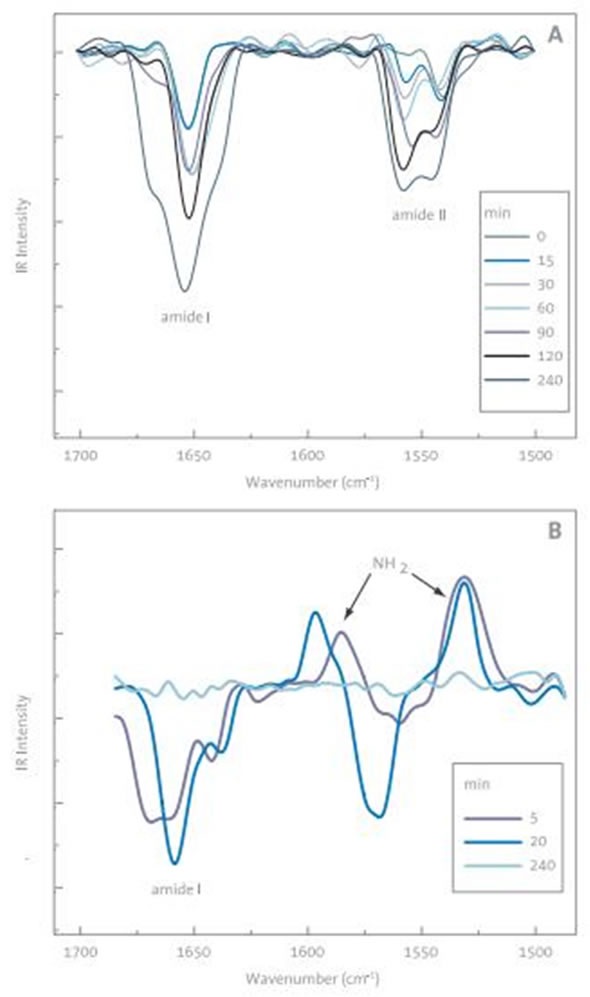
Figure 5. A) Change in the amide-region of PM-IRRAS spectrum of the BLG absorbing into DMPA monolayer with time. The initial surface pressure was 20 mN/m before BLG injection. B) Removal of BLG from DMPA monolayer when chitosan is injected to the subphase
BLG’s adsorption to the DMPA monolayer was examined using the PM-IRRAS spectrometer as a function of time (Figure 5A). The typical DMPA (CH2 scissor) peaks in 3000cm-1 region were found to be positive and the typical bands for amide II (NH bending), amide I (C=O stretch) and polypeptides were found to be negative.
During PM-IRRAS measurements of monolayers, resonance parallel and perpendicular to the surface are observed as positive and negative peaks, respectively. The development of the peak intensity over time supports the conclusion that BLG either adsorbs increasingly to the surface over time or undergoes reorientation. These findings are supported by other experiments performed on the system, such as fluorescence from deposited LB films and rheological measurements.
Conclusion
The two case studies above demonstrate how floating model membranes can provide extremely useful microenvironments for examining how biomolecular interactions may occur in real biological systems.
The Langmuir isotherm studies showed that chitosan and BLF both adsorb to a DMPA monolayer and that chitosan can remove BLG from the phospholipid layers that have a negative charge. The PM-IRRAS measurements provided evidence of the mechanism by which chatoyant removes protein.
About Biolin Scientific
Biolin Scientific is a leading Nordic instrumentation company with roots in Sweden, Denmark and Finland. Our customers include companies working with pharmaceuticals, energy, chemicals, and advanced materials, as well as academic and governmental research institutes. Our precision instruments help discover better drugs faster, develop better solutions for energy and materials, and perform research at the frontiers of science and technology.
Biolin Scientific proprietary systems are based on nanotechnology and advanced measurement techniques. They have earned leadership in several industries through our commitment to scientific excellence and continuous product development.
Our commitment to customer service and application support is a key feature of our operations. We focus on working together with customers and building long-standing relations. Today, Biolin Scientific provides products and services in more than 70 countries around the world.
Sponsored Content Policy: News-Medical.Net publishes articles and related content that may be derived from sources where we have existing commercial relationships, provided such content adds value to the core editorial ethos of News-Medical.Net which is to educate and inform site visitors interested in medical research, science, medical devices and treatments.