Biomedical applications of microspheres in drug delivery and bone tissue engineering offer many advantages over other particle geometries.
Microspheres can be made to have a uniform size and shape to enhance their delivery to target sites and to have a large surface area to enable sufficient therapeutic coatings.
However, their manufacture is associated with development and production challenges, especially in large-scale production.
Microspheres can be produced with different materials, including glass-, ceramics-, and polymer-based microspheres, which provides them with varying properties and applications.
Porosity
Porosity is an important consideration in microsphere production, particularly for tissue engineering and drug delivery applications. Porous microspheres enable a greater loading efficiency and improved control over the release of medications, growth factors and other components.
Their large surface area also means they are favorable for cell attachment and proliferation. Other important features to consider during porous microsphere production are interconnected and open porosity and favorable pore size.
Porous microspheres can be made with external and/or internal porosity and with or without interconnectivity for cell attachment and proliferation over the surface area.
Although porous microspheres possess these superior features, non-porous microspheres can be useful for certain applications that require higher mechanical properties such as bone tissue regeneration.
Polymer based microspheres
Polymer-based porous microspheres have been a significant focus of attention due to their potential in controlled drug release, either by degradation of the polymer matrix or through the leaching of drug components from the polymer.
During production, the selection of either synthetic or natural biodegradable carrier matrices for drug delivery is an important consideration. Natural polymers such as collagen and protein undergo enzyme degradation, whereas synthetic polymers degrade by hydrolytic activity.
The synthetic polymer polylactic acid (PLA) is a common polymer used for biomedical applications due to its degradation rate and desirable mechanical properties. Solvent and emulsion-solvent evaporation processes can be used to create microspheres with differing morphologies.
For example, in 1991, S Izumikawa and colleagues reported investigating progesterone drug-loaded poly (l-lactide) (PLLA) microspheres prepared using a solvent evaporation process.
They found that removing volatile solvent at atmospheric pressure formed PLA microspheres that were crystalline in structure, whereas at lowered pressures, the removal of the solvent led to the formation of microspheres with amorphous polymer matrices.
The researcher’s study suggested that the crystalline microspheres with rough surfaces and large surface areas enabled rapid drug release compared to the smooth, amorphous structures.
Protein microspheres are widely used as natural polymer microspheres in drug delivery due to their selective uptake by specific cells, their high biological activity and the numerous sites they provide for drug components to attach to. They can also be combined with numerous different drugs to produce derivatives with specific pharmacological properties.
Glass and ceramic based microspheres
Glass and ceramic-based spheres are generally studied for tissue regeneration, radionuclide therapy and applications in dentistry or orthopedics.
Glass based microspheres
The three types of glass-based microspheres are generally borate, silicate and phosphate-based.
Borate-based glass spheres, have become particularly attractive as delivery vehicles of biodegradable radiation, especially in the treatment of arthritis, owing to their uniform size and shape. These spheres were often considered ideal for radiation synovectomy (the removal of the synovium membrane that lines a joint).
The use of silicate-based bioglass, glass–ceramics microspheres and silica nanospheresin biomedical applications has also been investigated. A glass-ceramic phase is a material that has one or more crystal phases placed within a glassy matrix.
Various studies have concentrated on the generation of silicate-based microspheres, but achieving the correct size distribution and surface texture as well as avoiding aggregation has proved challenging.
One method used to manufacture microspheres is the sol-gel method via the Stöber process which was investigated by researchers Hui Liu and colleagues in 2012. Hydrolysing and polycondensing tetraethoxysilane ethanol solution (TEOS) has previously produced monodispersed silica microspheres 0.3µm in size.
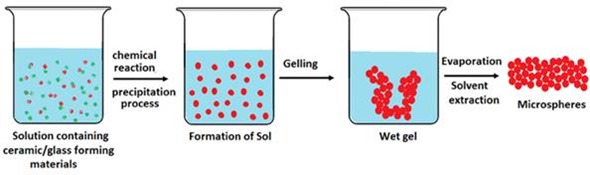
Scheme of production of glass microspheres via Sol–gel
Adding aluminum nitrate and silver nitrate resulted in 0.4µm microspheres coated in finer particles, which led to aggregation of the samples. Subsequent heating at 1000˚C resulted in microspheres 8.8 to 10.1 µm in diameter with smoother surfaces, but particles aggregated. The more aluminum nitrate and silver nitrate that was added, the more aggregation increased.
The antibacterial silver ions were only effective when the microspheres were initially submersed in ultrapure water, indicating that silver nitrate was only incorporated on the microsphere surface, therefore forming a short-term antibacterial shell.
The researchers then instead tried adding aluminum tri-isopropoxide to partially hydrolysed TEOS to form Si–O–Al bonds. Subsequent addition of silver ions to the ATIP/TEOS mixture in ammonia and silver nitrate solution, followed by centrifugation resulted in monodispersed microspheres, 0.4 to 0.6µm in diameter.
Subjecting the spheres to heat treatment did not change the diameters of the spheres or lead to their aggregation. The technique resulted in silver-doped microspheres that released silver ions in water in a much more controlled and gradual way than previously and these microspheres demonstrate great potential as antibacterial materials.
Ceramic based microspheres
Calcium phosphate-based ceramic microspheres are used in dentistry, orthopedics and pharmaceutics, because of their excellent osteoconductivity and biocompatibility.
Using ceramic microspheres, minimally invasive implantation can be achieved and ensures the target site receives adequate mechanical support. However, compared to glass microspheres, these materials are linked with high brittleness and slow resorption rates.
The spherical shape of these ceramic materials means they are thought of as more well suited for bone defect filling because of their packing and the predictability of their flow characteristics during injection, compared with particles of an irregular shape.
In at 2006 study, C.C. Ribeiro and colleagues made porous ceramic microspheres with interconnected porosity by mixing calcium-titanium-phosphate (CTP) and hyaluronic acid (HA) with a solution of alginate, the natural polymer found in brown algae and soil bacteria.
The ceramic phase-to-polymer ratio was critical to altering the size distribution of the resulting microspheres. Using a CTP ceramic-to-polymer ratio of 10:3 and 20:3, the mean microsphere diameters reported were 513 ± 24 µm and 602 ± 28 µm, respectively, whereas with HA the same formulation resulted in mean diameters of 429 ± 46 and 632 ± 40 µm.
Production challenges
Although production procedures have resulted in material-specific microsphere yields of favorable size and sphericity, some challenges still exist.
Mainly problems associated with large-scale production, since multiple steps are involved in the manufacturing process with high time and cost implications that make it difficult to scale-up the process.
The multiple steps involved in production can also cause changes to the spheroidised material. For example, the thermal processing required for the manufacture of silicate-based glass microspheres can result in crystallization of the glass, which can increase brittleness and affect degradation.
The production of ceramic-based microspheres may also be associated with high brittleness, complicated manufacturing processes and high production costs.
Acknowledgements
Produced from materials originally authored by Kazi M. Zakir Hossain, Uresha Patel and Ifty Ahmed. This has been adapted by materials provided by SpringerOpen original source can be accessed here: http://link.springer.com/article/10.1007/s40204-014-0033-8
The original article is distributed under the terms of the Creative Commons Attribution License which permits any use, distribution, and reproduction in any medium, provided the original author(s) and the source are credited.
References
- Cai Y, Chen Y, Hong X, Liu Z, Yuan W (2013a) Porous microsphere and its applications. Int J Nanomed 8:1111–1120. doi:10.2147/IJN.S41271
- Choi SW, Zhang Y, Yeh YC, Lake Wooten A, Xia Y (2012) Biodegradable porous beads and their potential applications in regenerative medicine. J Mater Chem 22:11442–11451. doi:10.1039/c2jm16019f Bohner et al. 2013
- Chen M, Wang X, Ye Z, Zhang Y, Zhou Y, Tan WS (2011) A modular approach to the engineering of a centimeter-sized bone tissue construct with human amniotic mesenchymal stem cells-laden microcarriers. Biomaterials 32:7532–7542
- Edlund U, Albertsson AC (2002) degradable polymer microspheres for controlled drug delivery. In: degradable aliphatic polyesters, vol 157 Advances in Polymer Science. Springer, New York pp 67–112
- Kohane DS, Tse JY, Yeo Y, Padera R, Shubina M, Langer R (2006) Biodegradable polymeric microspheres and nanospheres for drug delivery in the peritoneum. J Biomed Mater Res Part A 77:351–361. doi:10.1002/jbm.a.30654
- Hong S, Hsu HJ, Kaunas R, Kameoka J (2012) Collagen microsphere production on a chip. Lab Chip 12:3277–3280. doi:10.1039/c2lc40558j
- Bergquist NR, Holubar K, Diaz GA, Beutner EH (1972) The manufacture of protein microspheres by suspension polymerization. Int Arch Allergy Immunol 43:791–799
- Han Y, Radziuk D, Shchukin D, Moehwald H (2008) Stability and size dependence of protein microspheres prepared by ultrasonication. J Mater Chem 18:5162–5166. doi:10.1039/b807615d
- Jung T, Kamm W, Breitenbach A, Klebe G, Kissel T (2002) Loading of tetanus toxoid to biodegradable nanoparticles from branched Poly(Sulfobutyl-Polyvinyl Alcohol)-g-(Lactide-Co-Glycolide) nanoparticles by protein adsorption: a mechanistic study. Pharm Res 19:1105–1113. doi:10.1023/a:1019833822997
- Athanasiou KA, Agrawal CM, Barber FA, Burkhart SS (1998) Orthopaedic applications for PLA-PGA biodegradable polymers. Arthrosc J Arthrosc Relat Surg 14:726–737
- Waris E, Ashammakhi N, Kaarela O, Raatikainen T, Vasenius J (2004) Use of Bioabsorbable Osteofixation Devices in the Hand. J Hand Surg 29:590–598
- Fu HL, Rahaman MN, Day DE, Huang WH (2012) Long-term conversion of 45S5 bioactive glass-ceramic microspheres in aqueous phosphate solution. J Mater Sci Mater M 23:1181–1191
- Stöber W, Fink A, Bohn E (1968) Controlled growth of monodisperse silica spheres in the micron size range. J Colloid Interface Sci 26:62–69
- Ribeiro CC, Barrias CC, Barbosa MA (2006) Preparation and characterisation of calcium-phosphate porous microspheres with a uniform size for biomedical applications. J Mater Sci Mater Med 17(5):455–463. doi:10.1007/s10856-006-8473-x
- Martinelli JR, Sene FF, Kamikawachi CN, Partiti CSD, Cornejo DR (2010) Synthesis and characterization of glass-ceramic microspheres for thermotherapy. J Non Cryst Solids 356:2683–2688. doi:10.1016/j.jnoncrysol.2010.05.006
- Liu H et al (2012) Preparation of porous hollow SiO2 spheres by a modified stober process using MF microspheres as templates. J Clust Sci 23:273–285
- Kawashita M, Toda S, Kim HM, Kokubo T, Masuda N (2003) Preparation of antibacterial silver-doped silica glass microspheres. J Biomed Mater Res A 66A:266–274. doi:10.1002/Jbm.A.10547
About SpringerOpen
SpringerOpen, launched in June 2010, includes Springer’s portfolio of 200+ peer-reviewed fully open access journals across all areas of science.
In August 2012, due to the growing demand for open access and the success of our SpringerOpen journals, we expanded our offering to open access books. Published under the SpringerOpen brand they complement our established open access journal portfolio.
SpringerOpen journals and books are made freely and permanently available online immediately upon publication. They are subject to high-level peer review, author and production services ensuring quality and reliability of the work.
Authors publishing with SpringerOpen retain the copyright to their work, licensing it under a Creative Commons license. To cover the cost of the publication process, all SpringerOpen journals and books charge an open access fee.
To support the individual researchers in covering these costs, institutions can join our Open Access Membership Program which we offer together with BioMed Central. We currently have over 450 members in more than 45 countries.
Publishing with SpringerOpen enables authors to widen their readership, comply with open access mandates, retain copyright, and benefit from Springer’s trusted brand!