It is notoriously difficult to ensure precisely targeted drug delivery, with a range of variables impacting the efficacy of any given active ingredient. Tailored nanoparticles have been integrated into drug delivery applications to better address the limitations of traditional drug delivery, especially the challenges inherent to effective targeting and timed release.
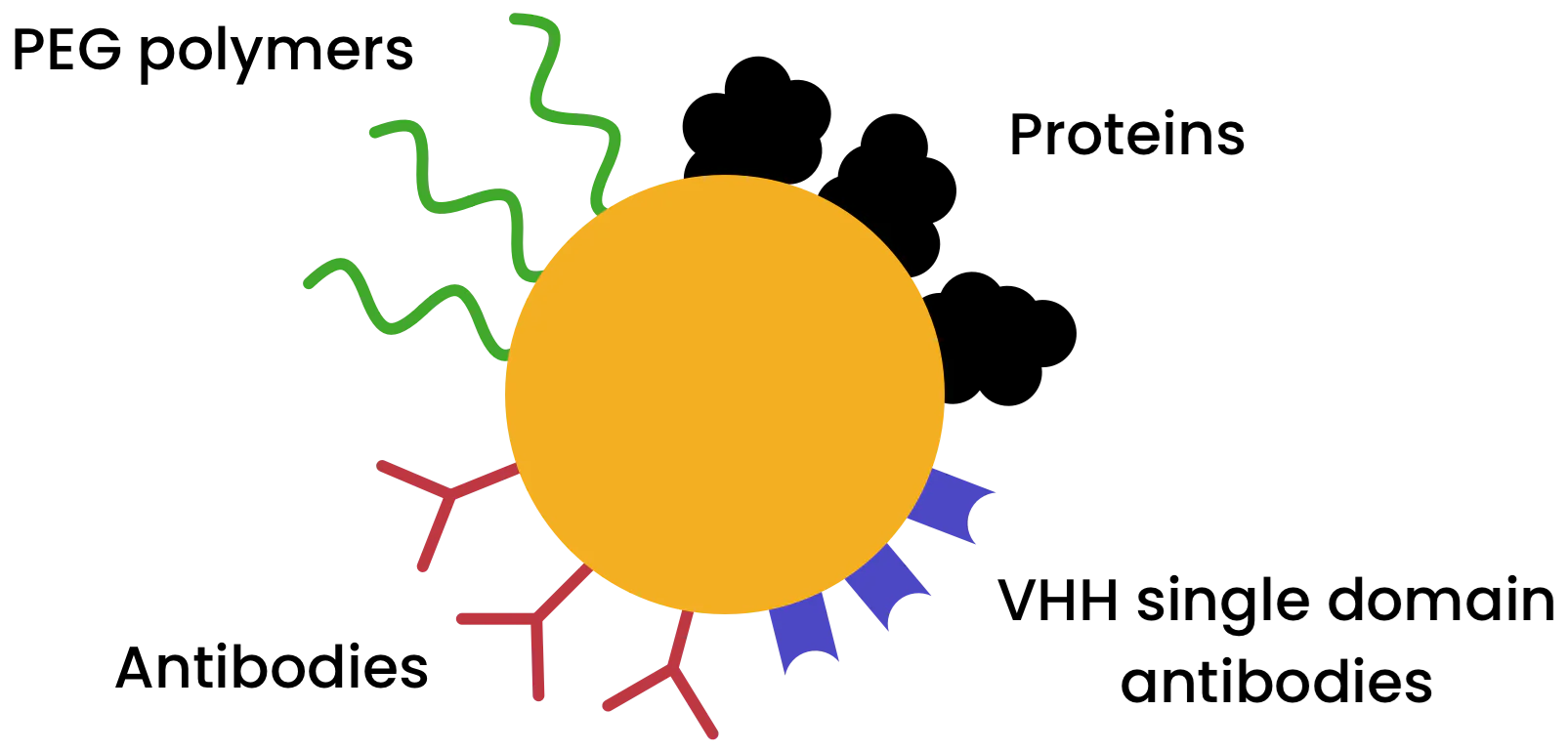
Image Credit: nanoComposix
When designing a nanoparticle-enabled drug delivery system, many intersecting considerations must be taken into account, such as the target location of the drug payload, the method of delivery, uptake kinetics, and circulation time.
Functionalization of the nanoparticle surface represents a key facet of this design process. Functional surface chemistry groups enable the attachment of specific targeting ligands designed to guide the nanoparticle to its target location within the body, for example, circulating biomarkers, organ-specific cell membrane proteins, or other disease-specific proteins.
Suitably designed surface chemistries are also key to ensuring that the nanoparticle can withstand its environment prior to delivery. These designs will differ depending on the delivery method in question, with different considerations for topical, intravenous, oral, or inhalation delivery routes.
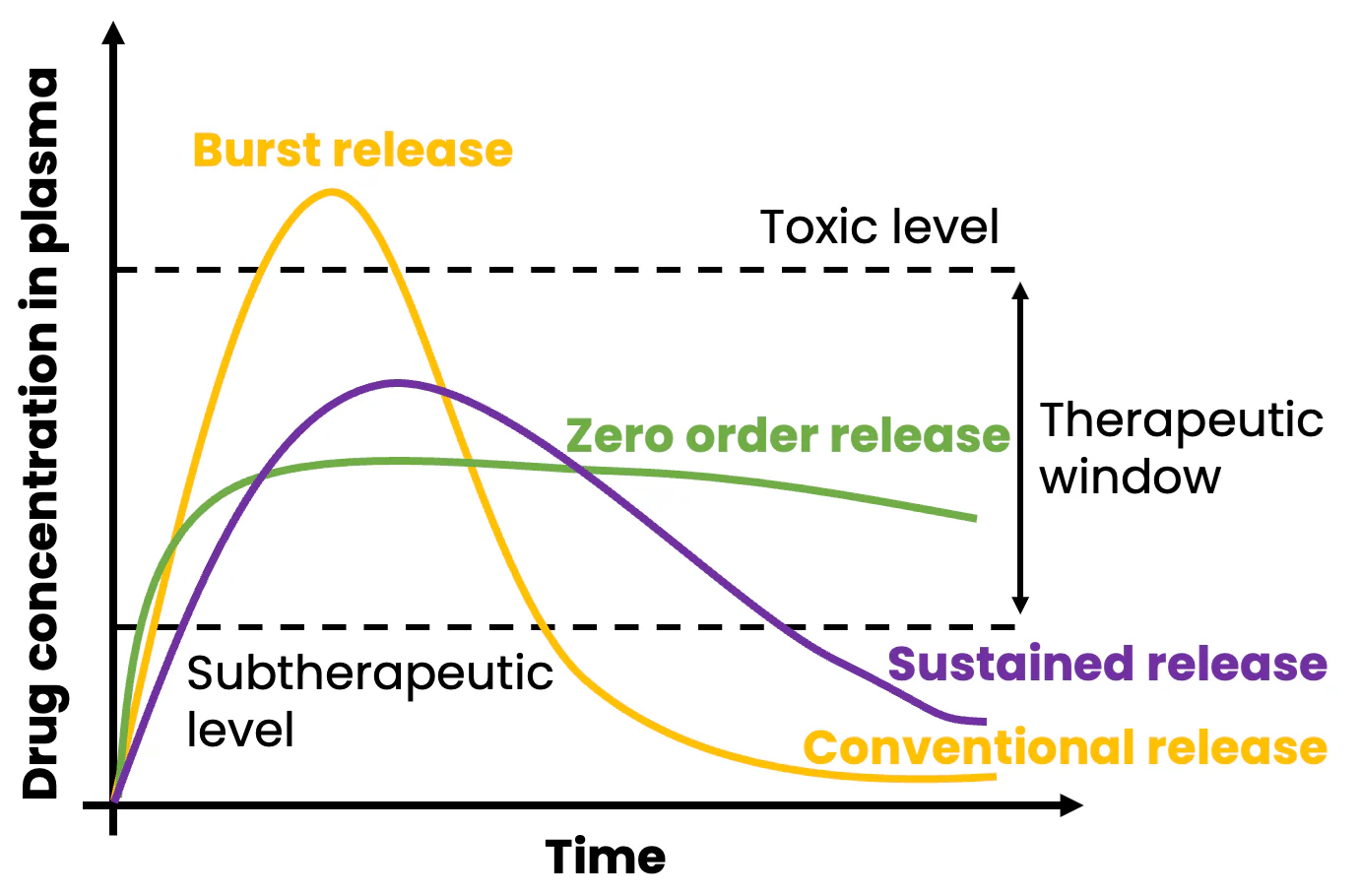
Image Credit: nanoComposix
A nanomedicine may be required to inhibit or promote enzymatic activity, deliver a therapeutic, induce apoptosis, upregulate or downregulate a particular pathway, or deliver genetic material.
It is important that the nanomedicine delivers the payload to the correct location and maintains the appropriate concentration for sufficient time (the therapeutic window) to allow the medicine to be effective.
To accomplish this successfully, the nanomedicine must be able to circulate in the body long enough to release its targeted drug payload and then maintain a therapeutic concentration long enough to achieve its goal prior to the immune system clearing the nanoparticle.
Nanoparticle quality by design (QbD) considerations for this application include several considerations. For example:
- Should the nanomedicine be administered once or multiple times?
- What are the required drug release kinetics in this instance?
- Do the nanoparticles accumulate in the body, or is there a specific clearance pathway to consider?
By adjusting the nanoparticle composition, shape, size, and surface functionalization, the nanomedicine's release, circulation, and clearance profiles can address these considerations.
This format offers a range of customization options for nanoparticle-based delivery systems. It also offers the option to encapsulate cytotoxic drug payloads at high loadings while effectively hiding their presence prior to their targeted delivery.
This format works by building on recently approved antibody-drug conjugates (ADCs) used as novel chemotherapeutic agents. For example, new therapies involve a monoclonal antibody (mAb) covalently attached to a drug molecule to enhance cytotoxic material delivery to tumors when contrasted against traditional administration routes.
Because only a small number of drug molecules can be attached to each antibody, ADCs suffer from relatively low drug loading. Using hollow or porous nanoparticles engineered to encapsulate the drug while targeting specific locations enables a highly concentrated payload to be delivered while reducing non-specific delivery, potentially improving therapeutic efficacy and patient outcomes.
Nanoparticle selection guidelines
A number of factors must be considered when selecting a nanoparticle type and surface chemistry for drug delivery applications, such as the delivery route, toxicity, and chemical properties of the drug to be loaded, the desired drug concentration in the nanomedicine, and the desired lifetime of the drug within the body.
Nanoparticle material and morphology
Silica nanoparticles
It is possible to reproducibly prepare solid or porous silica (amorphous SiO2) nanoparticles of various sizes while maintaining high uniformity. These particles can be modified via standard silane chemistry to introduce a wide range of surface functionalization options.
Silica is an inorganic material, but because it is a commonly occurring compound in biological creatures, it can be biodegraded and ultimately renally excreted. Its combination of biocompatibility and biodegradability has made silica nanomaterials the delivery method of choice for a diverse array of active pharmaceutical ingredients (APIs).
Solid silica particles exhibit nanopores that can incorporate low molecular weight species. Their surface can also be functionalized to be highly positively charged, allowing it to electrostatically adsorb genetic material, for example, to deliver silencing RNA to specific cells.
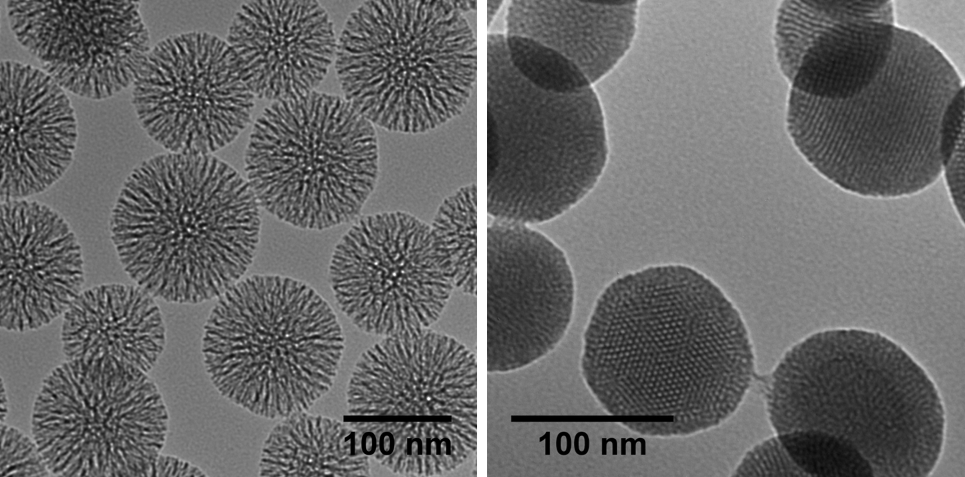
Image Credit: nanoComposix
Mesoporous silica nanoparticles (MSNs) can be used to ensure the delivery of larger drug molecules or higher payload loadings. These nanoparticles have garnered significant attention over the last 20 years, primarily due to their potential for versatile cargo loading and surface functionalization.
This family of biocompatible inorganic nanoparticles can accommodate an array of APIs, ranging from small hydrophobic drugs to large biomolecules. This is achieved by varying the size of the nanoparticles’ pores and leveraging selective surface modification, methods that can often be independently tailored to introduce diverse functionality either inside or outside their porous matrix.
Previous studies and applications have demonstrated a high loading capability of up to 30% by weight for small molecules in mesoporous silica, and it is not unusual for messenger RNA (mRNA) in large pore particles to reach 10% by weight.
It is also possible to incorporate other types of nanoparticles into the core of solid and mesoporous silica nanoparticles, creating composite particles with multiple functionalities. Particles can also be introduced to act as tracers.
For example, Silica shells with tunable thickness and surface chemistry may be deposited around gold cores. These particles see beneficial uses in pharmacodynamic, pharmacokinetic, and biodistribution studies, where the particles’ location and concentration may be determined by elemental analysis of gold, which does not naturally occur within the body.
Source: nanoComposix
Application |
Key Design Parameter* |
Advantage |
Visual Representation |
✓ Drug Delivery
✓ Catalysis
✓ Chemical Removal |
Hexagonal pore geometry |
Controlled molecular adsorption & release |
MCM-41** |
Negative (silica) or positive (aminated) surface functionality options |
Ability to perform specific chemical transformations |
|
100 nm particles with 3 nm pores |
Accommodates most small molecules & drugs |
|
Large pore radial geometry |
Accommodates larger APIs |
|
*Certain design parameters require a custom design
**Radial and cubic pore geometries also available as custom materials
Metal nanoparticles
Gold remains the most widely utilized metal for nanoparticle drug delivery and therapeutic applications. This is primarily due to its inert chemical properties and general biocompatibility.
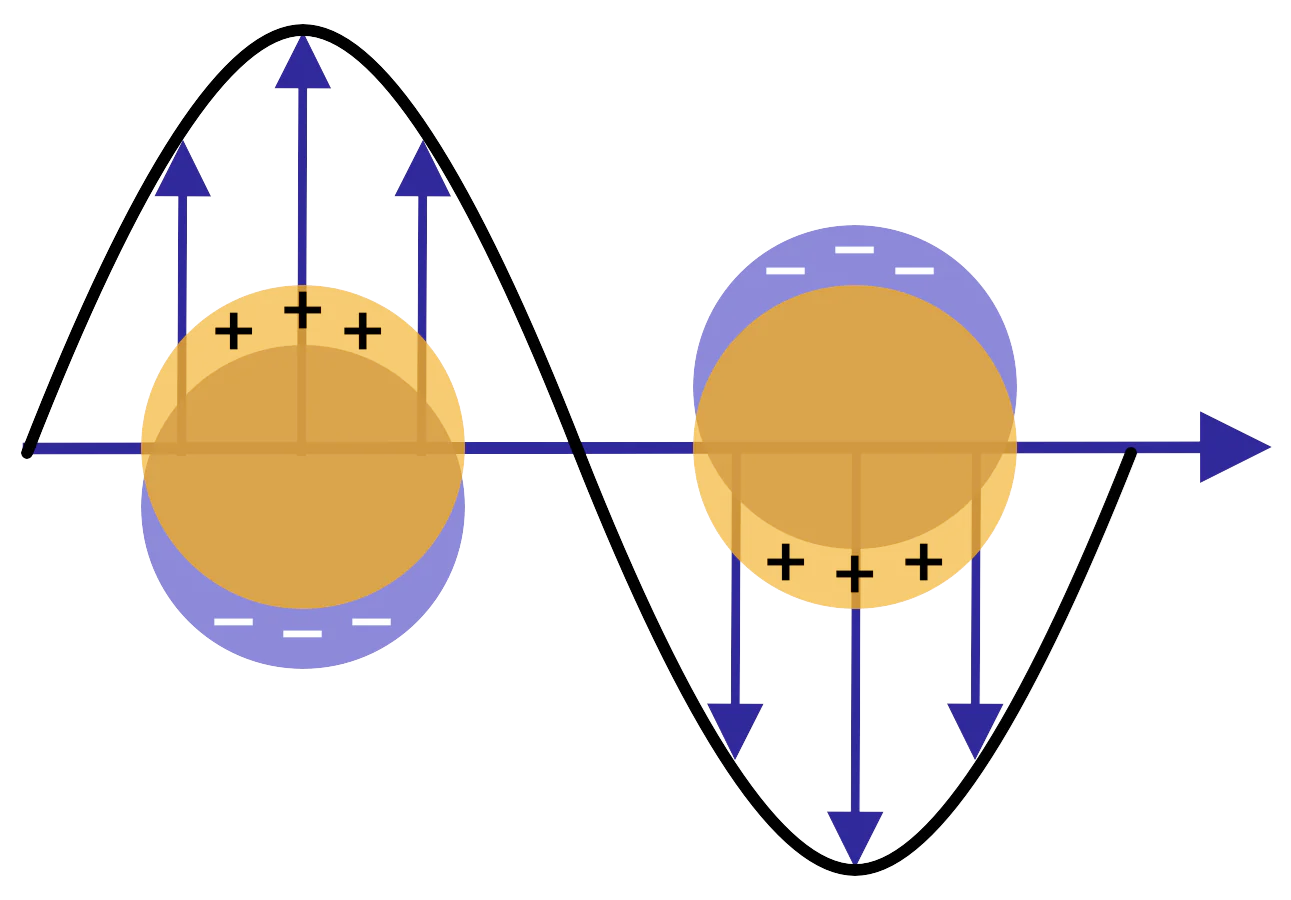
Image Credit: nanoComposix
Current applications of gold nanoparticles in nanomedicine leverage gold’s distinct optical properties, specifically the localized surface plasmon resonance (LSPR) that can be tuned from visible to near-infrared (NIR) wavelengths via alterations to particle size and morphology.
Gold-based nanoparticles can readily be utilized as theranostics agents in therapeutic and diagnostic spaces. They have also been employed in diverse applications, ranging from photodynamic and photothermal therapeutics to two-photon luminescence and cell identification via surface-enhanced Raman spectroscopy (SERS).
PLGA nanoparticles
Over the past few decades, nanoparticles fabricated from poly(lactic-co-glycolic acid) (PLGA) have become attractive vehicles for drug delivery. This has been primarily due to the material's biocompatibility and biodegradability and the resulting FDA approval of the polymer for therapeutic uses.
It is possible to fabricate this class of copolymer with different ratios of lactic and glycolic acids to fine-tune material properties and adjust the degradation rate in the body. The polymer’s terminal end groups can also be selected to create PLGA terminated with esters, amines, and carboxylic acids, offering significant nanoparticle design and functionalization versatility.
The synthesis route for the PLGA particles may be tuned to yield uniform particles in sizes between 100 nm and 1 µm, depending on the specific application needs. These characteristics enable the encapsulation of a wide range of APIs, including hydrophobic and hydrophilic small molecules, RNA, and proteins, with typical loadings up to 10 wt% of API.
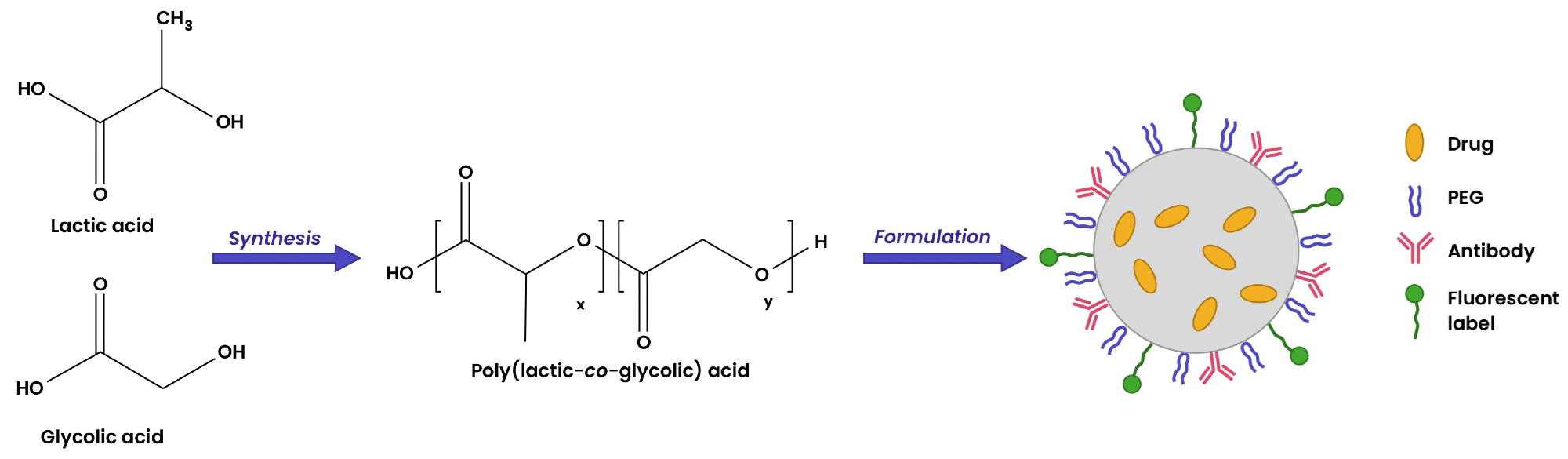
Image Credit: nanoComposix
Nanoparticle surface modification
The implementation of nanoparticles in drug delivery applications necessitates the selection of a suitable particle shape, size, and material type and the appropriate targeting ligands and surface chemistry.
nanoComposix can modify the surface of a wide range of nanoparticles with specific functional groups, biocompatible polymers, and biomolecules such as antibodies, proteins, and oligonucleotides.
The table below summarizes some of nanoComposix’s most commonly applied surface modifications for drug delivery applications.
Source: nanoComposix
Modification |
Description |
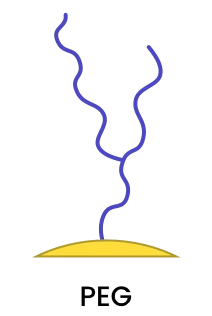 |
Polyethylene glycol (PEG) ligands offer a high degree of biocompatibility, can improve stability in high salt environments, and reduce non-specific interactions. PEG ligands can be covalently bound to the metal surface to produce stable coatings. In addition, certain bi-functional PEGs can be employed to introduce other surface functionalization. |
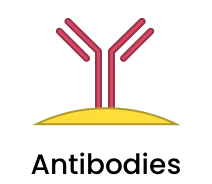 |
Antibodies (Ab), including fragments and VHH single domain antibodies can be conjugated onto a variety of nanoparticles using standard EDC/NHS chemistry or via a host of bioorthogonal click chemistry methods, depending on the particle type. |
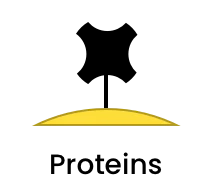 |
Proteins are able to be conjugated onto a nanoparticle surface using the same type of conjugation chemistry used for Ab. If more than one protein per nanoparticle needs to be conjugated onto a nanoparticle, this can be accomplished using bioorthogonal click chemistry. |
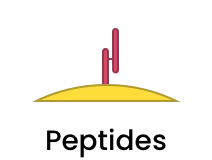 |
Sometimes only a short fragment is needed to direct the nanomedicine to the correct target, and can be accomplished by conjugating specific peptide sequences to the nanoparticle surface. |
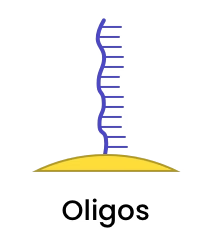 |
Oligonucleotides (oligos) can be covalently attached or encapsulated. |
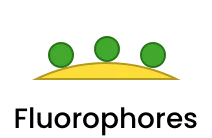 |
Various fluorophores can be covalently attached or may be encapsulated within the nanoparticle. |
Examples of drug delivery applications using nanoparticles
Triggered drug release using gold nanoparticles
Functionalized gold nanoparticles can release their payload in a target location via near-infrared (NIR) light irradiating, resulting in plasmonic heat generation.
Gold nanoparticles be augmented with a variety of compounds, including doxorubicin, polymers (PEG, poly(N-isopropyl acrylamide), hyaluronic acid, folate, proteins (ovalbumin, biotin), and DNA and RNA (siRNA and shRNA).
This versatility makes gold nanoparticles a versatile platform for delivering therapeutic cargo via the thermal stimulation of an array of heat-responsive coatings.
Silica and mesoporous silica nanoparticles
It is possible to engineer silica nanoparticles to specific sizes, and these nanoparticles can withstand enzymatic digestion and tolerate low pH. These characteristics have led to the employment of both solid silica and mesoporous silica nanoparticles in several oral drug delivery applications, with APIs' bioavailability increasing by 50 to 350% in these instances.
Their high surface area allows mesoporous silica nanoparticles (MSNs) to encapsulate a wide range of APIs at high loading levels. Both the pore size and total particle size of MSNs can be controlled. In some cases, the surface and interior of the particle may also be independently functionalized.
For example, a simple one-stop surface modification can allow genetic materials to be adsorbed on the outer surface of positively charged MSNs, while smaller drug molecules can be effectively trapped inside the pores.
When working with more sensitive materials such as messenger RNA, cavity size can be increased to accommodate their larger size and protect them from adverse environmental conditions.
Basic surface modification approaches can also be used to functionalize these particles with temperature, pH, or enzyme-responsive capping molecules to further direct the delivery of their cargo under certain triggered or prescribed conditions.
Silica’s inherent versatility allows the creation of composite core-shell materials, incorporating gold, iron oxide, or silver nanoparticles as cores surrounded by a uniform silica shell. This can facilitate the release of cargo upon irradiation and magnetic activation or be used to enable synergistic drug-metal cation effects.
PLGA
By adjusting the degradation rate of PLGA, researchers can control how quickly drugs are released. This is key to enabling sustained release and potentially more efficient drug delivery systems (DDS).
PLGA nanoparticles can also be modified at the molecular level to target specific tissues, further enhancing drug delivery and potentially limiting side effects in healthy cells.
The combination of this targeted approach and the ability to monitor drug delivery via imaging has revolutionized in vivo techniques for cardiovascular diseases, cancer, and central nervous system disorders.
References and further reading
- Buchman, Y. K.; Lellouche, E.; Zigdon, S.; Bechor, M.; Michaeli, S.; Lellouche, J.-P. Silica Nanoparticles and Polyethyleneimine (PEI)-Mediated Functionalization: A New Method of PEI Covalent Attachment for siRNA Delivery Applications. Bioconjugate Chem. 2013, 24 (12), 2076–2087. doi.org/10.1021/bc4004316
- Croissant, J. G.; Butler, K. S.; Zink, J. I.; Brinker, C. J. Synthetic Amorphous Silica Nanoparticles: Toxicity, Biomedical and Environmental Implications. Nature Reviews Materials 2020, 5 (12), 886–909. doi.org/10.1038/s41578-020-0230-0
- Danhier, Frederic, et al. "PLGA-based nanoparticles: An overview of biomedical applications." Journal of Controlled Release, vol. 161, no. 2, 2012, pp. 505-522. doi.org/10.1016/j.jconrel.2012.01.043
- Gao, Qinyue, et al. “Gold nanoparticles in cancer theranostics.” Frontiers in Bioengineering and Biotechnology, vol. 9, 2021. doi.org/10.3389/fbioe.2021.647905
- Ghitman, Jana, et al. "Review of Hybrid PLGA Nanoparticles: Future of Smart Drug Delivery and Theranostics Medicine." Materials & Design, vol. 193, 2020, p. 108805. doi.org/10.1016/j.matdes.2020.108805
- Janjua, Taskeen I., et al. “Clinical Translation of silica nanoparticles” Nature Reviews, Materials, 2021, 6, 1072-1074. doi.org/10.1038/s41578-021-00385-x
- Kennedy, Laura C., et al. “A New Era for cancer treatment: Gold‐nanoparticle‐mediated thermal therapies.” Small, vol. 7, no. 2, 2010, pp. 169–183. doi.org/10.1002/smll.201000134
- Liberman, A., Mendez, N., Trogler, W. C., & Kummel, A. C. “Synthesis and surface functionalization of silica nanoparticles for nanomedicine,” Surface Science Reports, 2014, 69(2-3), 132-158. doi.org/10.1016/j.surfrep.2014.07.001
- Liu, Ji, et al. “Gold nanorods coated with mesoporous silica shell as drug delivery system for remote near infrared light‐activated release and potential phototherapy.” Small, vol. 11, no. 19, 2015, pp. 2323–2332. doi.org/10.1002/smll.201402145
- Mir, Maria, et al. "Recent Applications of PLGA Based Nanostructures in Drug Delivery." Colloids and Surfaces B: Biointerfaces, 2020. dx.doi.org/10.1016/j.colsurfb.2017.07.038
- Mitchell, Michael J., et al. “Engineering precision nanoparticles for Drug Delivery.” Nature Reviews Drug Discovery, vol. 20, no. 2, 2020, pp. 101–124. doi.org/10.1038/s41573-020-0090-8.
- Moorcroft, Samuel C., et al. “Nanoparticle-loaded hydrogel for the light-activated release and photothermal enhancement of antimicrobial peptides.” ACS Applied Materials & Interfaces, vol. 12, no. 22, 21 2020, pp. 24544–24554. doi.org/10.1021/acsami.9b22587
- Popat, Amirali, et al. “Programmable drug release using bioresponsive mesoporous silica nanoparticles for site-specific oral drug delivery.” Chem Comm. 2014, 50, 5547-5550. doi.org/10.1039/C4CC00620H
- Saint-Cricq, P.; Deshayes, S.; Zink, J. I.; Kasko, A. M. “Magnetic Field Activated Drug Delivery Using Thermodegradable Azo-Functionalised PEG-Coated Core–Shell Mesoporous Silica Nanoparticles.” Nanoscale 2015, 7 (31), 13168–13172. doi.org/10.1039/C5NR03777H
- Saint-Cricq, P.; Wang, J.; Sugawara-Narutaki, A.; Shimojima, A.; Okubo, T. “A New Synthesis of Well-Dispersed, Core–Shell Ag@SiO2 Mesoporous Nanoparticles Using Amino Acids and Sugars.” J. Mater. Chem. B 2013, 1 (19), 2451–2454. doi.org/10.1039/C3TB20210K
- Tao, Yu, et al. “Light: A magical tool for controlled drug delivery.” Advanced Functional Materials, vol. 30, no. 49, 2020. doi.org/10.1002/adfm.202005029
- Vallet-Regi, M.; Rámila, A.; del Real, R. P.; Pérez-Pariente, J. “A New Property of MCM-41: Drug Delivery System.” Chem. Mater. 2001, 13 (2), 308–311. doi.org/10.1021/cm0011559
- Vines, Jeremy B., et al. “Gold nanoparticles for photothermal cancer therapy.” Frontiers in Chemistry, vol. 7, 2019. doi.org/10.3389/fchem.2019.00167
- Yang, Y.; Zhang, M.; Song, H.; Yu, C. Silica-Based Nanoparticles for Biomedical Applications: From Nanocarriers to Biomodulators. Acc. Chem. Res. 2020, 53 (8), 1545–1556. doi.org/10.1021/acs.accounts.0c00280
- Zhao Q, Sun X, Wu B, et al. “Construction of homologous cancer cell membrane camouflage in a nano-drug delivery system for the treatment of lymphoma.” J Nanobiotechnol 19, 8, 2021. doi.org/10.1186/s12951-020-00738-8
- Zhao, Q, Wu B, Shang Y, et al. “Development of a nano-drug delivery system based on mesoporous silica and its anti lymphoma activity.” Applied Nanoscience, 2020. doi.org/10.1007/s13204-020-01465-0
Acknowledgements
Produced from materials originally authored by Fortis Life Sciences.
About nanoComposix
Since 2004, nanoComposix has provided monodisperse and unagglomerated metal and metal-oxide nanomaterials to thousands of customers. Hundreds of different variants of material, size, shape, and surface are available as stock products and we have produced over 2000 custom core/shell, biofunctionalized, fluorescent, and magnetic nanocomposites to meet client specifications. nanoComposix produced the NIST nanosilver reference material and our particles have been utilized in over 2000 peer-reviewed publications. All of our materials are supplied with certificates of analysis that include electron microscopy, hydrodynamic diameter, and optical data for each batch to guarantee products meet specifications.
Sponsored Content Policy: News-Medical.net publishes articles and related content that may be derived from sources where we have existing commercial relationships, provided such content adds value to the core editorial ethos of News-Medical.Net which is to educate and inform site visitors interested in medical research, science, medical devices and treatments.