News-Medical talks to Professor David Thomas about his recent research identifying the mechanisms behind cancer cells developing resistance to drugs and how this new understanding could be used to pioneer better cancer therapies.
What led you to carry out this research?
The first reason is that we were very interested in thinking about drug resistance in a different way. Drug resistance is arguably the most significant challenge to cancer drug development at present. Most of the drugs that we have spent billions of dollars developing, and pay billions of dollars to provide to patients, are used in patients who are incurable, and they eventually fail.
In terms of thinking laterally about how to have an impact, it seems important to ask: what can we learn about why cancer drugs fail?
The second thing is that we were not after the relatively specific problem of how any individual drug fails. There has been a lot of research studying why, for example, drugs that target the epidermal growth factor receptor stop working because of the acquisition of a specific mutation that is resistant to the drug to which the patient was originally exposed.
That is not so much how resistance arises in each case, but the general mechanisms--if any--which increase the probability of resistance. We were more interested in whether there was a fundamental process across many drugs and applicable across many cancers which might drive the emergence of those specific resistance mutations.
A final reason is due to my thoughts back in 2001, a long time ago. The reason I wondered about this process of stress-induced mutagenesis was because I am a fundamental believer in the principles of Darwin, of natural selection, and that genetic diversity fundamentally drives evolution.
The theory was that the mutation process itself was largely passive and random, and it was the environment that applied the selected pressure which sculpted order, or apparent order out of chaos.
Reflecting on cancer in general, it is clear that mutations are really important. It is also clear that the cancers that we can observe in the clinic are extremely severely deranged in many cases, and it always used to puzzle me as to how cancers could tolerate that degree of ongoing genetic instability.
And so one thought that I had was that perhaps they did not have a constantly elevated background rate of mutation. Maybe what we were observing was a kind of historical record of a life trajectory where maybe, under some stressed circumstances, there was a pulse of very high mutation rate, and which then stabilized.
That rate of mutation stabilized, but now it had a completely new configuration of the cancer genome. If mutations are not fixed, random, and continuous, but rather they go in waves, what evolutionary biologists describe as saltatory bursts or spikes of mutability, then the next question is: would it not make sense if mutability itself comes at a fitness cost? And it clearly does.
That is how we use chemotherapies. They cause cancers to regress because we overload mutations. Using this, it makes sense that there would be a relationship between the selection process that Darwin posited and feedback onto the random mutation process so that organisms can tolerate a higher degree of mutation rate, but only when the payoff is there. This gives an escape route out of an otherwise intolerable circumstance.
The corollary of that is that in a perfect world, suppose an organism is now perfectly adapted to its environment, presumably any mutations that occur in that state can only be deleterious. There is only one way to go - down. In a perfect environment, mutation rates would be really low.
You have these two extremes. First, a hostile environment where even though mutations are nasty, in that circumstance it is the only way to survive. At the other extreme, you have a perfectly agreeable environment, in which case any mutation rate is probably not a good thing. It just leads to a disadvantage.
So given that concept, we have asked the question: is drug exposure in the cancer setting associated indirectly with evidence of increased mutation rates? That is how it all began.
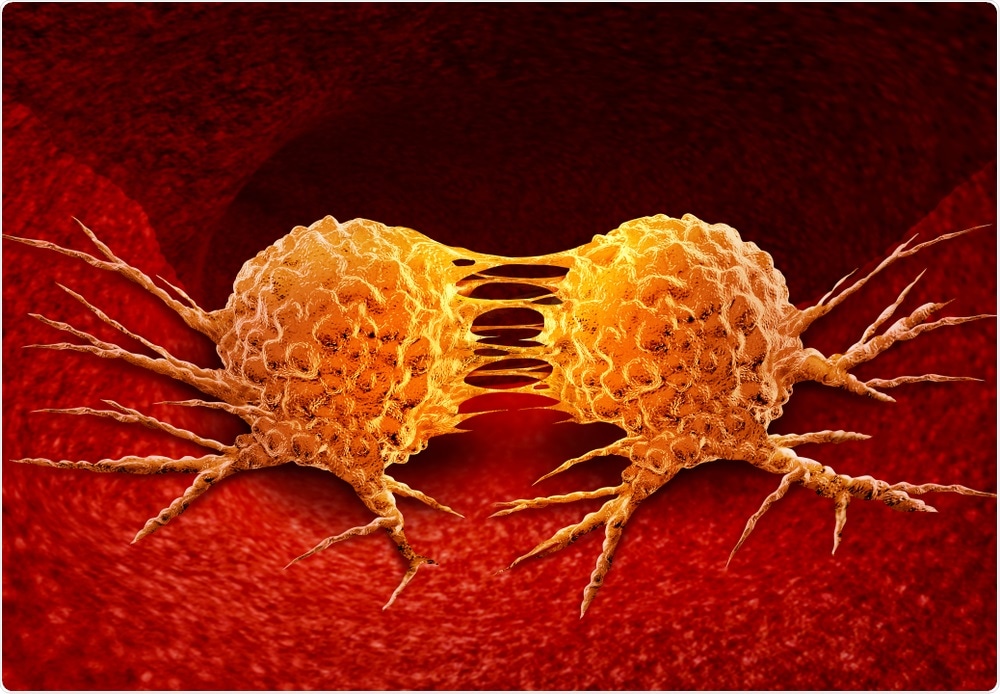
Image Credit: Lightspring/Shutterstock.com
What is stress-induced mutagenesis and how is it occurring in cancer cells?
Stress-induced mutagenesis is a process in a cancer cell, bacterium, or really any living organism, whereby exposure to a harsh or a hostile environment leads to an increase in mutation rates inside the cell's nucleus.
That general principle has been observed in bacteria and yeast. Factors like heat stress, nutrient deprivation, and exposure to drugs can cause it. We showed that if you took cancer cells and exposed them to a range of drugs, you could see a similar phenomenon - an increased rate of mutation inside cancer cells.
The whole study was about trying to understand the mechanisms by which that occurred. We were after something common to multiple different situations, rather than mechanisms that were specific to one drug and one cancer type. We wanted to find something relevant to multiple cancer types and different stresses.
The lead author of this paper, a wonderful researcher whose name is Arcadi Cipponi, asked the question: if we knocked out every gene individually in the human genome, would we find genes which were critical in common to the emergence of multiple types of resistance?
We called them facilitator genes because they did not directly lead to resistance, but they increased the probability that resistance would emerge. It turned out that the mTOR gene did this, which was a surprising result since it is actually an oncogene.
mTOR drives cancer cell growth, so the idea that knocking out mTOR would increase the chance of cancer surviving a drug was actually paradoxical. But we saw this in mTOR, amongst other genes. The other thing we know about mTOR, which made it a really fascinating candidate, is that it is quite evolutionarily conserved all the way down to yeast.
Yeast has a counterpart of the human mTOR gene, where it can be considered an evolutionary rheostat. This stress-sensing rheostat actually puts those organisms into what might be called a 'dauer' state, which is a sort of hibernation state that many primitive and lower organisms use to survive harsh environments. So it made a great candidate in this particular circumstance.
How does the stress-induced mutagenesis compare to that of bacterial resistance?
The specific pathways that we have uncovered in the range of cancers that we have looked at seem to hub on this mTOR gene. mTOR in bacteria does not appear to be as directly involved, but there are other systems that have been described that seem to coordinate stress-sensing mutagenesis in bacteria.
One of them, described perhaps 20 years ago, is called the SOS regulon. This is a sort of molecular circuit inside some bacteria that seems to increase the mutation rates in bacteria that are living in harsh conditions.
Normally we think of mutagenesis as being a copying problem, and that as the cells divide you get mutations randomly. Hence, if you increase the rate of division, you get more mutations. However, in this particular case, they were seeing stationary phase mutagenesis - as the cells started to slow down, they saw errors creeping into cells that apparently were not dividing.
This is really interesting because, if you think about it from an evolutionary point of view, if you are a sitting duck on a dish, not dividing because conditions are harsh, it is only by getting a mutation that you could possibly escape and regrow. So it makes sense that there should be a kind of survival mechanism like that in bacteria.
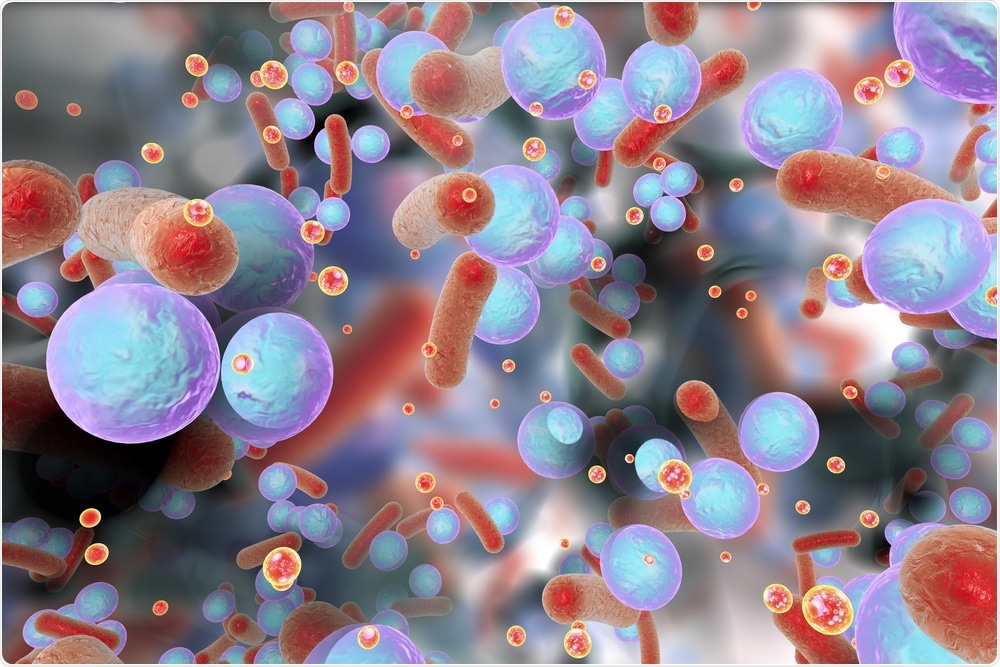
Image Credit: Kateryna Kon/Shutterstock.com
How did you go about investigating treatment resistance?
There is one experiment that I think is really important in this concept, which we did early on. We looked in human tissues exposed to drugs, human tumors from clinical trials, and our own culture systems, and showed this induction of increased mutation rates quite clearly.
In the key experiment, we took cells that were becoming resistant and had increased mutation rates, and removed the drug. Then we looked at how the cells were behaving without the harsh environment that was causing the increased mutation rate.
What we found was another paradox. The cells were doing better in the presence of the drug now, presumably because they were mutating, and therefore generating resistance mutations in populations of cells. These cells were capable of surviving where, for the most part, they would usually be rendered extinct by the drug. But when we took the drug away, the cells were limping. They were badly damaged.
This told us that, in the absence of selection, this mutation rate, or the processes that were going on inside the cell, posed a fitness penalty. The cells had acquired a disadvantage.
If you go back to the original idea, increased mutation rates should induce a fitness penalty because, for the most part, random mutations can only break something. It is a bit like if you take a watch, and it is ticking away perfectly well, but you hit it with a sledgehammer the likelihood is that it will not work better. It is probably going to break.
The corollary of that is also true; if you take a broken watch and you hit it with a hammer, the chances are it is not going to be fixed. However, there is a greater chance of a broken watch being fixed after being hit by a hammer than it is of starting spontaneously without any intervention. That is the kind of logic that is operating here.
So when we took those drugs away, we observed that these cells were struggling. That was a really important observation because not only did it suggest that under harsh conditions the cancer cells tolerated mutability and it gave them an advantage, but in the absence of that drug selection, it actually became a limitation on the cells.
That has two really big implications. The first is that resistance is not one process, it is two. You get the final product of the resistance mutation that causes the cells to no longer respond to the drug, but before that, there is a phase in which the cells are vulnerable because they have an increased mutation rate. That represents a therapeutic window of opportunity for combining drugs.
In the initial phase, there is a tension between the fitness penalty of mutation rates and the fitness advantage of generating resistant mutants. However, there is a longer phase during which the mutability dials back down so that the cells become stable once again in the new adapted configuration.
What we typically observe is the final process, but there is this period in between where there is a window of opportunity to do something that will stop the resistant cells from emerging.
What is mTOR, and why is it crucial to your findings?
mTOR is a critical hub of a growth pathway that acts as a sensor of conditions to the external world. It senses everything from nutrient deprivation to environmental stress, to acidity, to hypoxia, to the shutting down of growth factor pathways because of drugs.
Downstream of mTOR is the regulation of the whole cassette of genes that seem to be important for DNA repair. Once you inhibit mTOR, you increase the rates of mutation or the fact that mutations do not repair themselves. We observe that consistently across multiple cancer cell lines, and large databases, as well as in human systems.
The other thing about mTOR is that it is druggable. There are trials and drugs in the marketplace right now for cancer which target mTOR.
This is really important, because what we are saying is that if you silence mTOR, you increase the rate of resistance, and we have drugs that silence mTOR.
What we think is going on is that these drugs that target mTOR in the clinic have typically had the short term effects of shrinking the tumor, as you might expect, but we then observe objective responses and improvements in progression-free survival. You get a longer time until the tumor starts to grow. However, there has been a real dearth of studies that have shown an impact on overall survival.
What this suggests is that the tumors are catching up. After that initial response, drugs are actually losing the ground that they made in the early phase of the response to the treatment. And that could be because silencing that pathway accelerates the emergence of resistance, and adaptation, and fuels the progression and evolution of cancer to a more aggressive state.
While not a good thing, it does explain some interesting observations over the past 15-20 years in the use of mTOR antagonists, and antagonists of the upstream pathway, the PI 3-Kinase pathway, which have also had this property of leading to short term but not sustained benefits in overall survival.
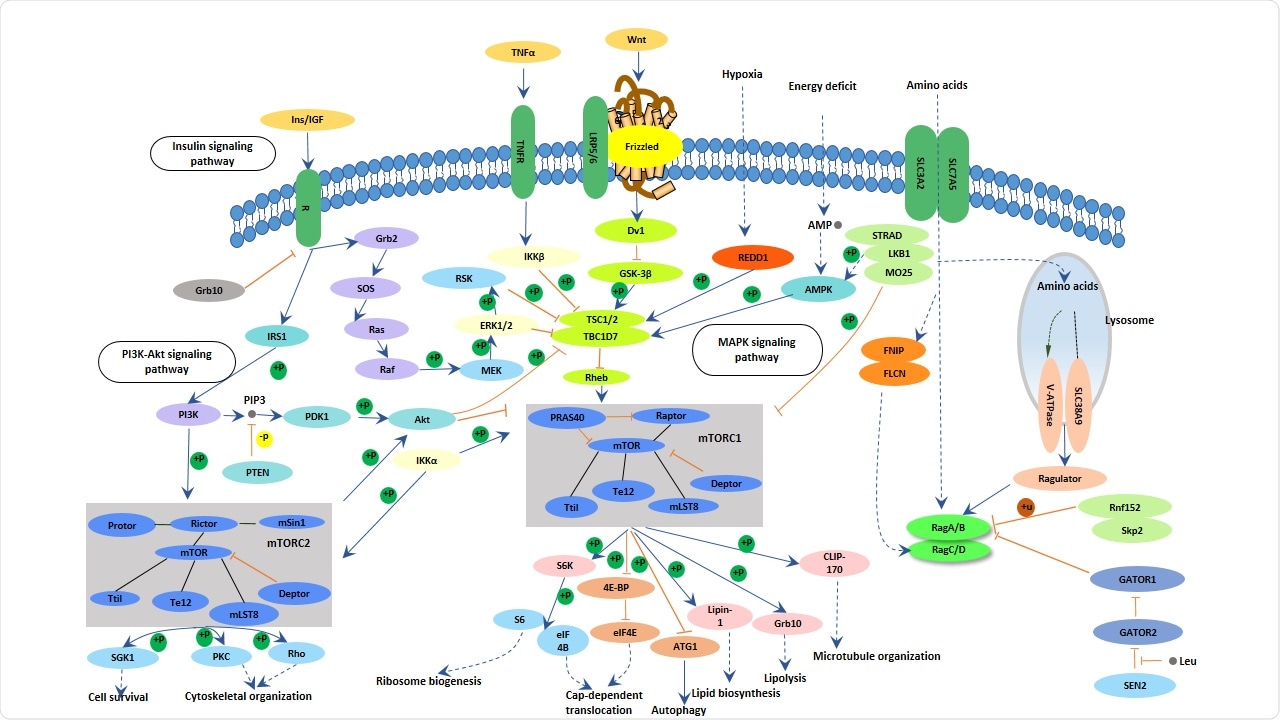
Image Credit: Cusabio.com
How was whole-genome sequencing used within your research?
We had to show there were increased mutation rates. To do this, we undertook whole genome sequencing of populations of cancer cells subject to drug selection, and what we were able to show is that we could see increased rates of emergence of all sorts of mutations in resistant clones, normalized for the number of cell doublings in those populations.
That is like sequencing a population of humans and looking at all their diversity. We were able to show, using whole-genome sequencing of this population, that there was increased diversity within resistant populations, but not in the original parental populations prior to exposure to drugs. We used it for this and to map those mutation patterns in more detail.
What cancers have you shown this mechanism to exist in?
We have seen suggestions of all of this in sarcomas such as liposarcoma, gastrointestinal stromal tumors, melanomas, breast cancer, and pancreatic cancer. Plus, colleagues who were working on a separate paper recently showed a similar thing in colorectal cancer.
This is one of the reasons why this mechanism is important. It is not just for one isolated cancer type, or one isolated target, or one isolated drug. It seems to be something that could be relevant to a lot of situations that cancer patients face.
How could this research lead to new therapeutic strategies and improve cancer survival?
This is a very important question. Think about a patient being exposed to a drug that induced this two-phase resistance process. What happens if, instead of just giving the drug on its own, you also give it with something that then increases the mutation rate further, taking the resistant cancer cells from a state in which they have an increased mutation rate which is still tolerable even though it is causing a fitness penalty, to pushing them over the edge?
That approach is called synthetic lethality. Some drugs exploit synthetic lethality in other situations already, which we can use to design studies.
That is what we are thinking of as the first therapeutic opportunity to exploit - to take a drug with a short-lived benefit, and, by combining it with a second drug that exploits the vulnerable phase of emergent resistance, to prolong its benefit.
This strategy depends on knowing that the first drug actually stimulates genomic instability, which is what our data has shown.
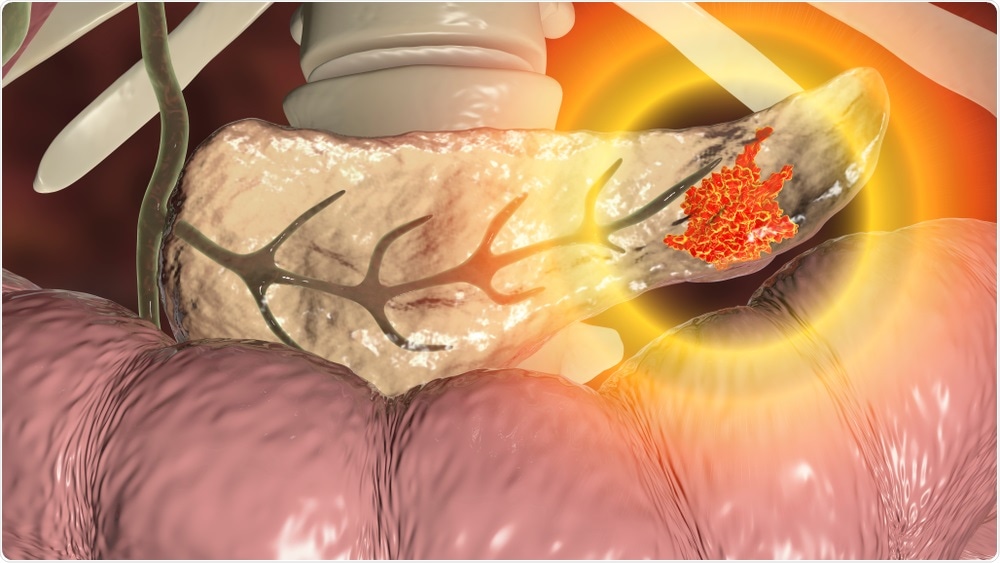
Image Credit: Kateryna Kon/Shutterstock.com
How did testing using a mouse model of pancreatic cancer support your theories with therapeutic improvement?
We tested this idea with a mouse trial. We took a human pancreatic cancer in what is called a xenograft, used it in a mouse, and treated it with a drug that we knew was not particularly effective on its own in the clinic. We combined it with one of these agents that should induce synthetic lethality.
Through this, we showed that you could increase the shrinkage of tumors much more effectively, and more importantly increase the survival of the mice, as we had predicted. It was, at least, the best preclinical evidence we could generate prior to conducting a trial.
What are the next steps in your research?
The obvious thing is to conduct a trial, and we are designing that trial right now. We are hoping to open that early next year.
We have a program at the Garvan called the MoST, the Molecular Screening, and Therapeutics trial. It is a signal seeking basket trial based around molecular biomarkers. We have been screening thousands of patients already, and we will screen another 3,000-5,000 patients over the next few years in that program.
What we are going to do is identify patients with the appropriate mutations, and then treat them with this combined approach that we have been discussing to see if the ideas that we have seen in the laboratory will translate into the clinic.
The second thing is that there are a whole slew of drugs being developed that target DNA repair and are looking for a place in the world. Those agents might well find their place as the second drug in combinations of the kind that we have been discussing. We think that there may well be different types of genomic instability, rather than just one type that kills drug resistance.
Although we have clearly demonstrated consistent patterns so far, what we would like to do is to go into it in more detail to see if we can work out the exact operation pathways and whether there are any subtle differences that may be important therapeutically. These might bring to bear logical decisions about accessing some of these newer drugs that target other aspects of the DNA repair pathway.
There is a bit of work to be done in getting a more detailed, more nuanced handle on exactly how the stress-induced mutagenesis plays out, and in particular, how it might relate to drugability.
Where can readers find more information?
Click here to read about the MoST clinical trials at the Garvan Institute of Medical Research: https://www.garvan.org.au/research/genomic-cancer-medicine-program/molecular-screening-clinical-trials
About Professor David Thomas
Prof Thomas is Head of the Cancer Theme at the Garvan Institute of Medical Research and Director of The Kinghorn Cancer Centre.
He is an NHMRC Principal Research Fellow and medical oncologist whose focus is on the application of genomic technologies to the understanding and management of cancer. Prof Thomas founded the Australasian Sarcoma Study Group, a national research organization, and established Australia’s leading adolescent and young adult cancer unit at the Peter MacCallum Cancer Centre.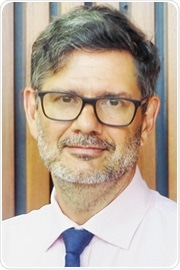
Dr. Thomas leads the International Sarcoma Kindred Study, now recruiting from 23 centers in 7 countries, and led the first international study of denosumab in Giant Cell Tumor of bone, leading to FDA and TGA approval.
He has over 150 research publications, including lead or senior author papers in Cancer Cell, Molecular Cell, Journal of Clinical Investigation, Lancet Oncology, JAMA Oncology, and Journal of Clinical Oncology. Since moving to NSW, he has established the Australian Genomic Cancer Medicine Centre, a national precision medicine program for patients with rare and early-onset cancers.
In 2018, he was President of the Connective Tissue Oncology Society, the peak international body in his field. He has created a national program in genomics medicine - the Australian Genomic Cancer Medicine Program - unifying for the first time the major cancer centers in every state and territory, as well as three of Australia’s leading research organizations (WEHI, Garvan, NHMRC CTC).