Animal survival relies on neural mechanisms that trigger behaviors to meet basic bodily needs. Traditionally, motivated behaviors have been categorized into three phases (Figure 1A; Craig, 1917).
The preparatory (foraging) phase is where a desired outcome is sought and obtained, and is often the result of an increasing physiological demand. The consummatory phase follows this, which consists of motor, reward, and sensory processes related to accomplishing the aims of the behavior (for example eating food or drinking water). During the consummatory phase, satiety builds and eventually stops the behavior, through the activation of PBNCGRP neurons for example (Campos et al., 2016, 2018).
The analysis of neuron dynamics is required to understand the neural processes for these motivated behaviors to establish the selective involvement of neurons in the satiety, consummatory, or preparatory phases. Signals of energy deficit activate the agouti-related protein (AGRP) neurons in the hypothalamic arcuate nucleus (ARC) (Cowley et al., 2003).
Studies, where AGRP neurons have been artificially activated in sated mice to generate a virtual need, describe how these neurons reflect aspects of hunger by initiating food-directed foraging and selectively inducing the avid consumption of food (Figure 1A; Aponte et al., 2011; Krashes et al., 2011).
AGRP neurons promote food-seeking, transmit negative valence, and are quickly repressed when food is located, which leads to activity being low during eating (Betley et al., 2015; Chen et al., 2015; Mandelblat-Cerf et al., 2015). AGRP neurons activate the preparatory stage of hunger, but they are not necessary for the consummatory stage of eating behavior.
Different neuron populations must be responsible for this phase if AGRP neurons do not perform a key role in prolonging food consumption (Sternson and Eiselt, 2017).
Motor circuits in the hindbrain regulate the rhythmic actions of ingestion (Wiesenfeld et al., 1977), but what regulates the length of food consumption and the control of ingestion is not fully known. The palatability of food is related to food pleasantness (hedonic value) and promotes a transition from ‘‘sampling to savoring’’ that extends consumption behavior (Yeomans, 1998).
Hunger increases subjective accounts of palatability in humans (Cabanac, 1971). The increasing prevalence of obesity may also be driven by simple access to cheap and highly palatable food (Johnson and Wardle, 2014).
Palatability is a main factor that influences appetite (Sørensen et al., 2003), but little is known about the relationship between the neural circuits through which hunger increases consumption and shifts palatability and the interoceptive sensory neurons.
Hunger is not the only factor that regulates ingestion. Thirst increases the consumption of water by nitric oxide synthase 1 (Nos1)- expressing neurons in the suprafornical organ (SFONOS1) that selectively promote water seeking, generate thirst-related brain state changes, and detect physiological dehydration (Allen et al., 2019; Betley et al., 2015; Oka et al., 2015; Zimmerman et al., 2016).
Thirst and hunger circuits related to SFONOS1 neurons and AGRP neurons are mainly considered as discrete interoceptive pathways for individual physiological states.
Less attention has been given to the behavioral mechanisms that are shared between these distinct physiological states and the possible convergence of thirst and hunger circuits.
To investigate this gap in the literature, the separately localized and molecularly defined SFONOS1 and AGRP neurons were used as entry points to evaluate the convergence of thirst and hunger circuits.
Glutamatergic neurons in the anterior peri-locus coeruleus (periLC) were identified as a polysynaptic convergence region in thirst and hunger processes. Causal perturbations and circuit dynamics demonstrate that periLC neurons regulate eating and drinking by controlling palatability during the consummatory behavior phase.
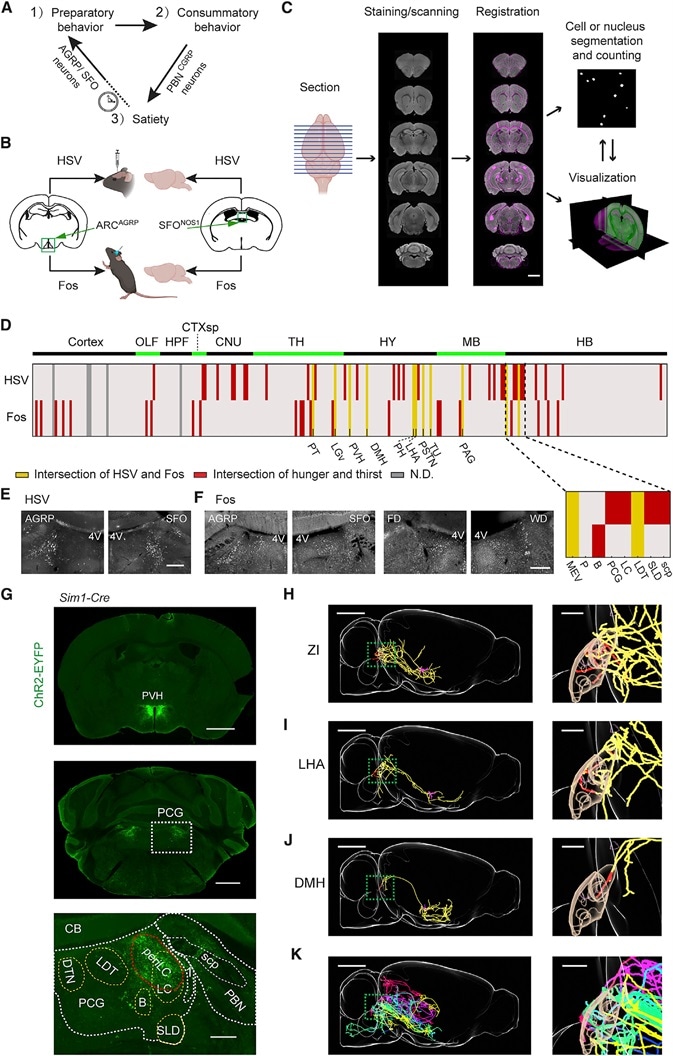
Figure 1. Brain Mapping Downstream of AGRP or SFONOS1 Neurons
(A) The three phases of motivated behavior.
(B) Intersectional strategy for anatomical (HSV) and functional (Fos) polysynaptic mapping of circuits downstream of AGRP or SFONOS1 neurons.
(C) Pipeline for data acquisition and registration to the Allen Institute Mouse Brain Reference Atlas. Scale bar: 1 mm.
(D) Intersection of labeling from HSV or Fos mapping across AGRP groups and SFONOS1 groups. Inset: 8 brain regions in and around the PCG. Red: anatomical or functional intersections. Yellow: four-way intersections. Gray: not determined. Abbreviations: see STAR Methods.
(E) HSV-tdTomato labeling in PCG. 4V: fourth ventricle. Scale bar: 500 mm.
(F) Fos-IR in PCG following AGRP or SFONOS1 neuron activation, food deprivation (FD), or water deprivation (WD). Scale bar: 500 mm.
(G) ChR2-EYFP expression in PVHSIM1 neurons (top, scale bar: 2 mm) shows axon projections in the PCG (middle, scale bar: 1 mm), and overlapping periLC (bottom, scale bar: 250 mm).
(H) Sagittal view of a neuron in ZI projecting to PCG. Magenta: soma and dendrites. Red: axons in the MEV (magenta mask), B, PCG, LC, LDT, and SLD (brown mask). Yellow: axons in other regions. Scale bar: 2 mm. Inset: PCG, scale bar: 500 mm.
(I and J) The same as in (G) with neurons from DMH (I) and LHA (J).
(K) Overlay of six additional neurons projecting to PCG: LHA (green), HY (blue), LHA (yellow), LHA (purple), ZI (red), and AHN (lake blue).
Image Credit: TissueGnostics
Results
Convergence of feeding and drinking circuits
SFONOS1 and AGRP neuron axon projections intersect in multiple brain regions downstream (Figure S1A). It was hypothesized that hunger and thirst circuits overlapped at certain points to regulate the shared processes of these behaviors.
A strategy was developed to execute a wide search for functionally important and anatomically-defined convergence points through the use of polysynaptic circuit mapping techniques (Figure 1B).
The research team searched for downstream brain regions where AGRP and SFONOS1 neurons intersected, which was detected by Cre-dependent herpes simplex virus (HSV) anterograde polysynaptic labeling (Lo and Anderson, 2011) with the fluorescent protein tdTomato.
Fos-mapping was also performed after SFONOS1 or AGRP neurons were optogenetically activated in the absence of water and food (Figures S1B and S1C) to locate downstream regions that are functionally activated in thirst and hunger.
From each group of mice, brains were stained, sectioned, and registered to the Allen Institute Reference Brain Atlas. Image segmentation of nuclei and labeled cells was then performed and Fos-immunoreactivity (IR) or HSV-mediated tdTomato expression were measured across several brain regions (Figure 1C; STAR Methods).
Multiple subcortical regions included both Fos-IR and HSV-mediated tdTomato expression, along with some of the direct downstream projection targets of SFONOS1 and AGRP neurons, for example paraventricular thalamus (PVT), lateral hypothalamic area (LHA), and paraventricular hypothalamic nucleus (PVH) (Figure 1D; Figures S1D–S1F). These have been found to be essential for various elements of hunger and thirst behavior in previous studies (Betley et al., 2013; Leib et al., 2017).
The researchers were particularly interested in whether this strategy could locate poorly characterized brain regions that play a role in eating and drinking, and a hotspot of Fos and HSV labeling was identified inside of the hindbrain at the dorsolateral area of the pontine central gray (PCG).
In both SFONOS1 and AGRP groups, similar labeling was found with HSV in the MEV (midbrain trigeminal nucleus), LC (locus coeruleus), PCG, SLD (sublaterodorsal tegmental area), LDT (laterodorsal tegmental area), and SCP (superior cerebellar peduncles) (Figures 1D and 1E).
FosIR was identified for both groups in the MEV, LDT and Barrington nucleus (B) (Figures 1D and 1F). Cells in dorsolateral PCG were also found to exhibit increased Fos in water-deprived (WD) mice or food-deprived (FD) mice but not in control mice (Figures 1F and S1G).
Along with this, the findings concurred with results of a recent study (Li et al., 2019), which showed that the PVH, which includes second-order neurons in thirst and hunger circuits (Atasoy et al., 2012; Leib et al., 2017), projects to the dorsolateral PCG (Figure 1G).
This region has been anatomically defined as the periLC (Shipley et al., 1996) and covers the brain region which surrounds the LC’s anterior portion, which is flanked dorsally by the fourth ventricle, medially by the LDT, and laterally by the MEV (Figure 1G; see STAR Methods for explanation of anatomical terms).
Single neuron reconstructions (Winnubst et al., 2019) demonstrated inputs to PCG that came from several brain regions related to ingestive behaviors (Figures 1H–1K). From these results, periLC and surrounding locations were investigated for their role in the regulation of food and water consumption.
Acknowledgments
Produced from materials originally authored by Rong Gong1, Shengjin Xu1, Ann Hermundstad1, Yang Yu2, and Scott M. Sternson1 from Janelia Research Campus1 and Allen Institute2.
About TissueGnostics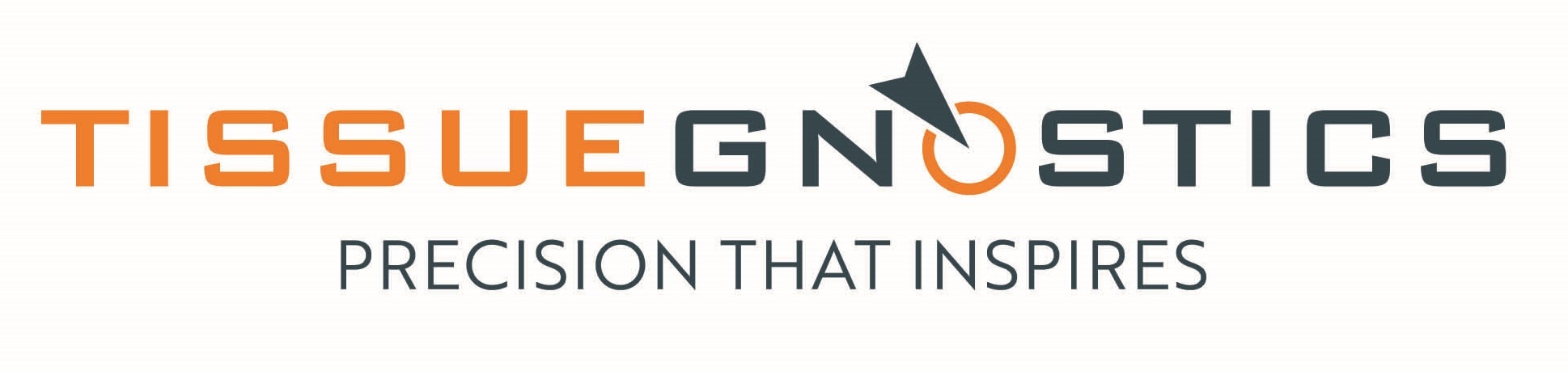
TissueGnostics (TG) is an Austrian company focusing on integrated solutions for high content and/or high throughput scanning and analysis of biomedical, veterinary, natural sciences, and technical microscopy samples.
TG has been founded by scientists from the Vienna University Hospital (AKH) in 2003. It is now a globally active company with subsidiaries in the EU, the USA, and China, and customers in 30 countries.
TissueGnostics portfolio
TG scanning systems are currently based on versatile automated microscopy systems with or without image analysis capabilities. We strive to provide cutting-edge technology solutions, such as multispectral imaging and context-based image analysis as well as established features like Z-Stacking and Extended Focus. This is combined with a strong emphasis on automation, ease of use of all solutions, and the production of publication-ready data.
The TG systems offer integrated workflows, i.e. scan and analysis, for digital slides or images of tissue sections, Tissue Microarrays (TMA), cell culture monolayers, smears, and other samples on slides and oversized slides, in Microtiter plates, Petri dishes and specialized sample containers. TG also provides dedicated workflows for FISH, CISH, and other dot structures.
TG analysis software apart from being integrated into full systems is fully standalone capable and supports a wide variety of scanner image formats as well as digital images taken with any microscope.
TG also provides routine hematology scanning and analysis systems for peripheral blood, bone marrow, and body fluids.
Sponsored Content Policy: News-Medical.net publishes articles and related content that may be derived from sources where we have existing commercial relationships, provided such content adds value to the core editorial ethos of News-Medical.Net which is to educate and inform site visitors interested in medical research, science, medical devices and treatments.