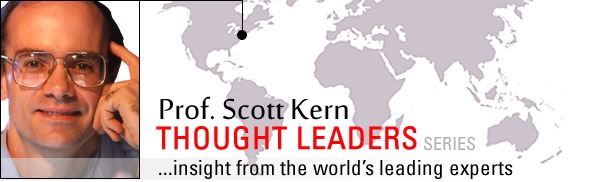
Please can you give a brief overview of mitosis and how abnormal mitosis can be linked to cancer?
Mitosis is the process by which a cell gives rise to two daughter cells. It’s the basis behind the growth of the human body, the renewal of the human body as it ages, and unfortunately, the growth of cancers.
Abnormal mitosis has been linked to cancer as far back as the late 1800’s by a man named David Von Hansemann.
Back then they didn’t even know that hereditary material in cells helped guide their behavior. They didn’t know that the chromatin was the hereditary factor, but they had suspicions, and David Von Hansemann noticed that there were abnormal mitotic activities in cancer cells.
Usually during mitosis, two poles are set up in the cell, like a North Pole and a South Pole of the Earth, and from these two poles there is an organization that pulls the genetic material, which we now know is in the chromosomes, to each of the poles, establishing two new polar centers of chromosomes and allowing two daughter cells to form from those two poles.
What David Von Hansemann noted at about 1880 was that cancers often have multipolar mitosis, in other words, an abnormal form of mitosis.
Not much was done with his observation until Theodor Boveri popularized it and expanded it considerably in a very focused monograph published in 1914. That’s the work, because it was published in English about 16 years later, which gathers the most attention when people talk about the mitotic abnormalities of cancer.
What Theodor Boveri proposed was that these multipolar mitoses in cancer were actually the cause of the chromosome abnormality and that they therefore contributed to the cause of cancer.
So the idea that these mitotic abnormalities were causal, and not just caused by cancer, was the key focal point around which there’s been a lot of research and speculation for the last 99 years. We’re nearly at the 100th year anniversary of Theodor Boveri’s proposal.
People have repeatedly confirmed David Von Hansemann’s observation of multipolar mitosis in cancers being much more common than in non-cancerous tissue. However, they’ve not been able to address the obvious questions raised by Theodor Boveri’s proposal: is multipolar mitosis a distinctive mechanism that we can understand, and does it actually help cause cancer?
Your recent research looked at a little-described gene known as FAM190A. How did you come to study this gene?
Well, there are two ways to decide to study a gene. One is: the scientists can decide to study it, and the second is: the scientists can just open their eyes and ears and see and look and listen to what the tumor cells are telling them to study.
Both have been successful over time, but perhaps the most fail-safe way to do this is to allow the tumor to tell you what to study, and in essence, the tumors were telling us to study this gene.
The clues that the tumors gave us were that the tumors accumulate mutations in certain genes. We then interpreted that the tumor accumulates mutations in these genes because these genetic mutations gave a survival advantage to the cells later misbehaved and became cancer.
As for the genes that don’t get mutated, we assumed that mutations also formed in those genes, but those cells did not gain the survival advantage.
Rather than assume that the tumor knows where it wants to go and puts mutations in bad genes, we assumed the opposite; that it obtains random mutations in virtually any gene, and the mutations that we see accumulating over time are merely because of the selective advantages that those cells have.
And so, when we noticed an accumulation of mutations within the FAM-190 gene, we suspected that it may have a causal role in the cancers. The original mutations that were noted by us and by a few other observers were deletions right in the middle of the gene. These were very strange types of mutations.
For instance, imagine a train with distinctive cars on it. Every time the train crashes, you notice that parts of cars are never destroyed, only entire cars, and that the remainder of the cars are connected up perfectly again, so the train can move fine but it may be missing cars five through seven. Meanwhile, cars up to car four and cars from car eight on down to the caboose are fine; they are connected together and work.
That’s what we noted in this gene. The cars in this analogy are the exons of the gene; they’re separated by something we derogatorily have been calling “junk” DNA, which are the introns.
In producing the final message of the gene, which is the protein—it’s the final bit of information that the gene produces, and it’s the business end of the gene—these exons are connected together to make an RNA, which has all these joints between the exons, and then a protein is made from those joined exons, which in my analogy are like cars of the train.
So if all the exons are joined together so that protein can be read from it, it is the same thing as a train being perfectly connected together and able to move. So the ribosome moves down this RNA, making a protein, and as long as the reading frame is not disrupted, it makes the protein nonetheless.
Almost always in cancer, when there are deletions of a gene, it rips up parts of exons or takes out the “lead engine car,” it takes out the “caboose,” or somehow it disrupts the gene so thoroughly that it’s unreasonable to think that the protein actually has a function.
In the case of this particular gene though, it did something that rarely occurs—it does occur, but rarely occurs—in other genes and cancers. It almost always removed groups of exons, so that when you add together the nucleotides, it was a multiple of three.
Since the reading frame is read in threes, it meant that the reading frame was still intact. In the analogy of the train, the disrupted cars are entirely knocked out; no other cars were damaged, and the train was connected up perfectly.
And so we suspect that there are altered forms of this FAM-190 protein that are made in tumor cells, and these must convey some kind of survival advantage, because these mutations occurred so similarly in a number of tumors.
Once we started looking beyond the deletions of the DNA, we found that there were even ten times more abnormalities of the exact same type when we looked at in the RNA. This could mean that we have not done a thorough job in looking at the original DNA; it could also mean that there’s another process that creates deletions in the RNA.
But this is our set of clues telling us that the tumors are sending us a signal: please examine this gene if you wish to understand how I became a cancer.
What did your research involve and what were your findings?
The most recent research was the first attempt by scientists to look at the function of this gene.
The FAM genes are fairly interesting. They’re in the back room, so to speak, of genetics. In fact, they all were named with the FAM prefix because the only thing you could say of any intelligence about these genes was that they formed a family. In some cases it was a family of one gene, sometimes a family of two, and the most trivial differences identified a family.
So these are the most meaningless families you could ever imagine, and in fact, as they found functions for some FAM genes, officials have changed the nomenclature and dropped that name and gave it a more proper, more informative name. FAM-190A in fact recently became renamed as CCSER-1.
This was apparently taken from the phrase “Coiled Coil Serine Rich One,” for it has a lot of serine.
Another thing that’s characteristic of these FAM genes is they have no recognizable domain structure. 90% of the time when a scientist looks at a sequence of amino acids, he’ll recognize it: the pattern of a kinase or a structural protein or some kind of nuclear localization signal … something is recognizable as a hint of a function.
Often there are many of these hints, and we call each of these ‘domains’. Domains tend to work in a certain way, so we actually know an awful lot about 90% of proteins just by looking at the domain structure. In the case of the FAM gene, that’s just not true.
There’s a considerable portion of the human genome which has not been assigned any genetic function, even though we know they are genes. It’s kind of remarkable—this is the back room.
FAM-190 had no recognizable domain structure. It has a lot of serine in it. It has something that is somewhat like a “coiled coil,” but it’s such a poor example that I think you can get into quite a debate about whether it actually is a coiled coil. And even if it was, we don’t know what it does.
So this particular paper tried to look at the basic functions that are likely for this protein. In order to do so, we had to essentially try to do everything. We determined protein localization, that is: where is it located in the cell? We looked at protein phosphorylation to find out: do these serines or other structures on the protein become phosphorylated? This is a major way by which the cell regulates proteins.
Further we looked at the protein regulation—in this case, the amount of protein, the apparent size of the protein and phosphorylation. We looked at how this form of protein modification was regulated and changed during the cell division cycle (where one cell becomes two).
The cell cycle is a fairly well-defined process that has many stages. We were able to look at the individual stages of the cell division cycle and find out how the protein was modified, and we think those are clues to how the cell regulates the protein.
We found that it changed its apparent size, its phosphorylation and its quantity as it went through the cell cycle. So the cell apparently is trying to control the amounts of FAM190A protein, and it often controls it during mitosis.
In fact, much of the protein is phosphorylated and then apparently destroyed in mitosis. This, for a biologist, sets off all kinds of memories of other interesting proteins which get destroyed during mitosis, some of which play a role in mitosis.
In addition, we looked at the effect of what happens when you make a deficiency of this gene, specifically a deficiency of its RNA and its protein. We also conducted a “knock-down” technology, using something called siRNA technology, and we were able to produce populations of cells that have stable, reduced amounts of this protein.
We think that if the protein were absent, the cell wouldn’t survive. So we were happy to have living populations that had a decreased amount of the protein.
In those populations we saw some very bizarre things that we made movies out of.
The last two things we did were to make antibodies against the protein, and we also did something called a yeast two-hybrid analysis, which is a way of getting a list of protein-protein interactions.
We found that the FAM-190 protein seemed to interact with other proteins that were important in mitosis. So here the tumor is telling us to look at it, and the various protein function tests that we were doing were telling us specifically to look at mitosis.
Now I’ll tell you a little bit about these movies and what we found in the culture when we tried to reduce the amount of protein in the cell.
When you reduce the amount of protein in a cell you start to figure out: why does the cell need that protein in the first place? It’s like kicking a chair out from underneath someone in a bar and you realize they needed it to remain up and off the floor.
The same thing was the case with this protein. We could make a deficiency of this protein and try to infer what role the protein had in the cell. And what we found were occasionally cells becoming either binuclear or multinuclear. This is something we occasionally see in some tumors, too.
It was Von Hansemann’s observations that it was fairly common, and it was Boveri’s proposal that it may be a causal feature that caused the cancers. Other scientists have since then observed that the tumors with abnormal chromosome amounts called aneuploidy have high amounts of multipolar mitosis, and the tumors that don’t have aneuploidy—and about 10% of all human cancers do not have aneuploidy—those tend to have very low amounts of multipolar mitosis.
Again, you still don’t know the chicken and the egg question, which came first? Did multipolar mitosis cause cancer, or do 90% of cancers cause multipolar mitotic properties?
What we found in this culture was the imposition of multipolarity on this population that otherwise had extremely rare multipolar cells. When we took movies of it we could see what was happening, and this is the hilarious part.
In the literature of multipolar division, binuclear cells or even chromosomal abnormalities such as tetraploidy, where you have twice the normal number of chromosomes, most of the experimentally imposed conditions only double the amount of chromosomes. Tetraploidy is easy to achieve experimentally, but much more than that is not.
Also, in many cancers we see chromosome numbers up to about tetraploid, but it’s hard to get numbers much above that for a large population of cancer cells. And in multipolar mitosis, we sometimes see three or four poles, but it’s difficult to see much more than that.
In our knockdown cultures, we saw something that was rather extreme. We were seeing something we occasionally do see in human tumors, where you get a cluster of bizarrely multinuclear cells and they seem to have maybe four or eight nuclei stuck together, maybe more. And that’s what was happening in these cultures, in occasional cells. This was an episodic event, we think if it was more common the culture would have died.
Specifically, it was an episodic event where a cell would try to divide, having the normal two poles during mitosis, and it would seem to form two cells. But if you looked very, very closely, there was still a strand of cellular material connecting them. So in actual formal terms we call this a failure of the last stage of mitosis, cytokinesis: cyto- meaning cell and kinesis meaning moving, where the two cells actually move away from each other.
What we were seeing was, they were trying to move away from each other, but they were still stuck together. There was still a strand of cytoplasm connecting these cells.
And then at the time of the next mitosis, they entered mitosis, kind of as expected, but now they had four poles, the chromosomes would come together and would try to separate into fourth cells. So now you have what looks like four cells that were all connected by strands of cellular material, cytoplasm.
And then they would all get together and say, “Certainly the third try’s going to work; let’s try to break apart into eight cells,” and they’d try that, and they had eight poles. During mitosis, all nuclei go to the respective corners, so to speak, and you have eight cells that are somewhat connected.
And then they’d say, “Let’s try one more time,” and by now you can’t count them in a microscope because some of the nuclei are sitting on top of each other and poles are sitting on top of each other. So you have something that might be somewhere near 16 poles trying to separate.
So now we’ve addressed the question: why don’t tumors (going back to my analogy) have loss of a caboose? Why don’t the tumors perhaps have loss of the engine at the front of the train? Why do we always have nice neat loss of just a few of the cars of the train? And the train still works. It’s still all connected; it’s still intact.
Well, we think it’s because if you really destroyed the gene fully, and it lost its function, you would have a cell that is so bizarre during mitosis that it could not possibly grow up to become a cancer. It could not grow up to become anything, not even a tissue. And it would probably die after a while. So this is our proposal, something we hope to study in the future.
Tumor cells still have the intact train of the FAM-190 gene but are missing a few cars. Are they sustaining subtle mitotic defects, that if more severe would kill the cancer or the emerging cancer, but if they were yet more subtle, would force the cancer to take an awful long time to develop?
In other words, it may like Goldilocks: just right, just enough chromosomal abnormalities to allow the cancer to experiment with bad genotype, the kind of genotype that would allow it to not obey the normal social rules of the tissue.
That’s our proposal, that when we study the types of FAM-190 abnormalities that are actually in the tumors, we’re going to find that it’s a subtle mitotic abnormality. That’s for future work.
The link between abnormal mitosis and cancer was discovered nearly a century ago by German scientist Theodor Boveri. Why has it taken so long to identify the common gene alteration that causes cancer-linked mitosis?
There’s been very little attention to multipolar mitosis. There have been people who studied it in association with kinases such as the aurora kinases and also other parts of that regulatory system such as polo kinases. But in general, when alterations in these kinases create abnormal mitotic
structures, the cells still tend to behave rather normally. In other words, they still tend to set up bipolar mitoses most of the time.
So, the first problem was that the experimental situations didn’t produce cells that were perhaps convincingly abnormal enough. The second problem was that these abnormalities of mitosis that had been found in cancers tended to be fairly low frequency; in other words, if I had a hundred tumors, they might affect five or ten of them.
A third problem was: many of the mitotic abnormalities of cancer that have been figured out affect the mid-portion of mitosis. That’s well after all the poles become separated. So they can’t explain why cancers have multipolar mitosis, because they explain abnormalities that come well after that point in the cell division cycle.
Meeting with these three difficulties, we’ve always missed the target. We’re shooting at the right place, but each of the bullets happened to have missed.
When you look at the cancer literature, very few people actually study late mitosis. Now, I don’t know why. It could be that until we understood the major regulations in the middle and early portions of mitosis, people just weren’t quite ready to get around to the last part of mitosis. It’s like eating your dessert before your hors d’oeuvre.
It could also be that studies of cytokinesis are a little hard to perform in human tissue. You see the two nuclei, you see the two bodies of cells; you assume that mitosis has proceeded normally. Well, you might not see strands of cytoplasm connecting those two cells showing that late stages of mitosis are actually not quite completed; in other words, that cytokinesis has failed.
So for a number of reasons which are hard to put your finger on, not enough people were shooting at that target, and when they did shoot at mitosis, they tended to miss the problems of cytokinesis.
So I would summarize that by saying it was unpopular. Most really new ideas of science have come from things that have been unpopular, and they can be unpopular because the techniques didn’t work out or because there wasn’t enough literature, but anytime you look at something that’s really cool, like these movies, you say, “Why didn’t anybody else see this?” It’s because it was unpopular.
Scientists don’t agree on what is popular, but certainly, some things are more unpopular than others, and late mitosis has been fairly unpopular to study.
It’s fairly unpopular with the funding agencies, not funding it even when applications are sent in requesting funding. How much progress there will be in this area, I don’t know. The U.S. government has increasing difficulties to fund medical research. Maybe private philanthropy will fund research on late mitosis; I really don’t know.
How common do you think alterations in FAM190A are and how do they compare to alterations in the more well-known genes such as p53 and p16 (also known as multiple tumor suppressor 1)?
If you look at the genetic level, it’s a little difficult to compare. P16’s a very small gene, p53 is only slightly bigger, and it’s fairly easy to get the perfect answer as to whether they have mutations or not when you look at them in tumors.
FAM-190 is nearly a million bases long. It is, if I would hazard a guess, about 40 times bigger than p53 gene.
And so, it may be a while before we know how often there are mutations in the DNA. We’ve already found about 4% of pancreas cancers to have deletions at the DNA level.
What many people do when the gene is really big and complex, like the FAM-190 gene or other genes, is they look at RNA and ask, is it disrupted?
And if you look at that level, the p16 gene and p53 gene are abnormal in about 50% of tumors and the FAM-190A gene is abnormal in about 40%, and the RAS gene is abnormal in about 20%. So that puts it in the top four.
What impact do you think your research on FAM190A will have?
In my dreams, I’d like to see that samples of people could be analyzed for abnormalities of this gene.
We do that in pathology labs around the world already for some other genes, and I think that this could be added to their panel of tests. That may aid in diagnosis and classification.
The second one is something we discussed in our first paper, which is that the abnormalities of this gene, because they leave the train intact so to speak and the protein is still made although it has deleted joints that are reconnected, allows you to propose that these joints are antigenic. An immune response could be raised against the joint.
In other words, you may have a cancer-specific antigen being formed. And that raises the possibility of an immunotherapy approach in which you try to develop a cocktail of immunoreactivities that would help to attack a person’s cancer.
Some years ago we used to say this with a lot of warnings, that it may never be shown to work. But actually there are cancer patients currently receiving immunotherapy. We’re seeing tumors shrink.
Others have received vaccines have not had recurrences, when they might have been expected to.
It’s no longer unreasonable to dream that you could use these joints that are in the FAM-190 gene at the site of the healed deletions, so to speak, the reconnected cars, and direct an immunotherapy against them. We plan to do that. This was recently funded, at a modest level, by the U.S. Government as part of a grant we have.
The third is something for the future that we’re very excited about. We’ve been exploring it a little bit, but we would like to more in the future. This is the idea that cells that have an abnormality in mitosis might be specially-susceptible to certain drugs. A normal cell with normal mitosis is probably resistant to all kinds of adverse influences, but a cell that’s fairly struggling to make it through a normal mitosis might be much more sensitive.
To do this you would have to understand, how do drugs affect cells that have a deficiency in late mitosis? That’s not been well studied yet. There are a few hints from defects studied in earlier stages of mitosis, but the cytokinesis stage has not been well explored for the types of drugs that would be seen to have a hypersensitivity.
Hypersensitivities are very important. For instance, when we treat a person with chronic myelogenous leukemia (CML) with Gleevec, the cancer cells are hypersensitive to it.
The rest of the body sees it as a toxin, no doubt, but they’re not hypersensitive to it in the ways that the cancer cells are.
The ability to elicit hypersensitivity reactions in cancer cells is what targeted therapy is all about. You first have to have a hook to grab on to on the cell; you have to know the cell has a very distinctive abnormality that you might be able to profit from by taking advantage of that abnormality.
Here we’re saying you might be able to profit from any mitotic abnormalities the gene might cause. We are still speaking in speculative terms about my fantasy for the future. I don’t know this to be true yet.
Do you think it will be possible to restore the activity of FAM190A in cancer patients in the future?
Simple: no, I don’t think so. It’s hard to fix something that’s broken. It’s easier to reduce something that’s overactive. For example, feedback mechanisms can reduce the overactivity of a certain protein. But it’s very hard to find a targeted therapy that actually turns something on that’s broken. Well, I’m not sure that it’s a reasonable dream.
But haven’t scientists recently been developing drugs to restore the activity of p53?
Scientists have been trying that with great success for 16 years. That should tell you something.
The idea’s now looking quite plausible for a subset of p53 mutations, but I’ve been following p53 since I had some big papers on the subject back around 1991 or 92, and we keep getting excited, but it has been a slow slog, so to speak.
I was once told, whenever you see a lot of papers on how something works or how something could work, it’s because nobody’s ever gotten it to work. So I’m cautious, but I wish them luck. Most disrupted genes, however, do not have the subtle mutations found in p53.
What are your further research plans?
We hope to tie together a speculation with some data to find out if this particular set of mutations in the tumors actually does what we’re proposing that it does.
In other words, we have puzzle pieces that look like they could fit together. Here, we have a protein involved in mitotic function. Here we also have a lot of mutations in the genome. We have even ten times more alterations in the mRNA in this gene.
All these puzzle pieces look like they should fit together into a picture. We’d like to put these puzzle pieces together to see if our proposal is correct.
Some people have misquoted us in the last few days, saying that we’ve established that FAM-190 causes mitotic abnormalities in cancer. No, what we did is find the missing puzzle piece that we hope fits in this puzzle.
Sometimes you find a missing puzzle piece and it goes to a different puzzle. It happens to me in my house all the time!
We think that these puzzle pieces probably fit together, and we’re proposing that, if we have the opportunity, we could generate a story whereby abnormalities of FAM-190 in cancers cause a subtle mitotic defect, and this creates antigens, it creates a diagnostic tool, and it creates a target for drugs that will elicit a hypersensitivity and kill the cancer cells more than they kill normal cells.
So that’s the kind of puzzle we’re trying to put together, and I think we have pieces that might fit together.
Where can readers find more information?
They can read our paper in The American Journal of Pathology: http://www.journals.elsevierhealth.com/periodicals/ajpa/article/S0002-9440%2813%2900280-0/abstract
About Professor Scott Kern
Dr. Scott E Kern is a leading scientist studying the molecular basis of human pancreatic and biliary cancers. He is co-director of the Gastrointestinal Cancer program of the Sidney Kimmel Comprehensive Cancer Center at Johns Hopkins. According to citation services, Dr. Kern has over 20,000 citations of his work among scientific publications, the majority concerning his pioneering studies of pancreatic cancer. His publications and citations place him among the top 1% of scientists in productivity and acclaim.
Dr. Kern’s laboratory was the first to accurately document genetic alteration of, localize, and publish sequences of the tumor-suppressor genes BRCA2 and DPC4. Due in part to their efforts, the genetic basis of pancreatic cancer is among the best-defined of all human cancers. They reported the genetic causes of various types of inherited cancer susceptibility, and they have found a very high number of specific genetic alterations in each tumor studied. Their studies of the precursors of the cancers established the principles of the new unified theory of pancreatic ductal tumorigenesis. With the possible exception of colorectal cancer, no other tumor system is known in greater detail. The research is accomplished in with a full spectrum of approaches, from the clinical interests of the world’s most active pancreatic cancer center to the application of cutting-edge basic science in a powerful, collaborative, multi-specialty approach.
Dr. Kern’s laboratory is currently developing drug screening systems to identify promising therapies for the common adult-onset cancers based on our understanding of BRCA2 and other disrupted DNA-repair functions in cancer cells. Signaling pathways of key tumor-suppressor genes are also explored in detail in order to suggest rational therapeutic approaches. His discoveries have been the basis of a number of US patents and novel and ongoing therapeutic trials for pancreatic cancer at Johns Hopkins and other centers. Recent publications have also addressed the presence of DNA-damaging activities in foods and flavorings.
Dr. Kern received his MD and undergraduate degrees from The University of Michigan in Ann Arbor. He was a graduate of an accelerated 6-year program that combined undergraduate and medical studies. He did initial research training and his residency at Michigan and became board certified in anatomic and clinical pathology. He joined The Johns Hopkins Cancer Center working with Dr. Bert Vogelstein’s molecular genetics group while completing a three-year fellowship in the pathology and molecular genetics of gastrointestinal cancer. In the early 1990s he teamed up with Dr. Ralph Hruban and with the surgeons of Johns Hopkins to begin studying pancreatic cancer. The select James S McDonnell Foundation supported his laboratory start-up. Soon, in 1996, his new team had identified the first example of human Smad gene, DPC4, which they found to be one of the major mutational targets of pancreatic cancer, and which was later discovered as the gene for a familial pediatric syndrome, juvenile polyposis. His group mapped the location of, and published the first sequence marking, the BRCA2 gene, whose mutations cause inherited forms of pancreatic, breast, and ovarian cancers.
Dr. Kern has won considerable recognition for his work. His 1996 paper in Science became the most-cited cancer research paper of that year. Dr. Kern was the laboratory co-chair of the NCI Progress Review Group for Pancreatic Cancer in 2000-1. He has served as an editor and reviewer for a number of journals and for scientific funding agencies. In 2004, he received the Louis A. Cochet Award for Outstanding Pancreas Cancer Research. The Thompson Essential Science Indicators in 2007 listed Dr. Kern as the most-cited laboratory researcher in pancreatic cancer. In 2010, he assumed a newly created endowed chair at Johns Hopkins, The Everett and Marjorie Kovler Professorship in Pancreas Cancer Research.