In this interview, Francesco Simone Ruggeri from the University of Cambridge talks to News-Medical Life Sciences about their research into biomolecules and the methods that are helping to achieve spectra at the single-molecule scale.
What first led you to studying chemistry and biology together?
I am a physicist, and I graduated in Italy with a Masters in solid-state physics and material science to characterize photovoltaic materials, so very different from my current research in bioscience. Once I had graduated, I decided that I wanted to study applied problems in physics, starting a Ph.D. at Lausanne in Switzerland and studying protein misfolding and aggregation using atomic force microscopy and general applied physical methods. Here I focused on studying the complex problem of neurodegeneration.
My research was at the interface between physics and biology. The physics part involved pure atomic force microscopy and biophysical methods, and the biology focus involved studying the molecular basis of neurodegeneration. After my Ph.D., I moved on to the University of Cambridge in the UK, working at the Centre for Misfolding Diseases where about 100 scientists from very different backgrounds – from biology to physics and chemistry – were studying the problem of protein misfolding and aggregation, which is considered to be one of the fundamental processes related to neurodegeneration.
Overall, my path started with physics, then I moved into biology and later into spectroscopy, working with chemistry along the way.
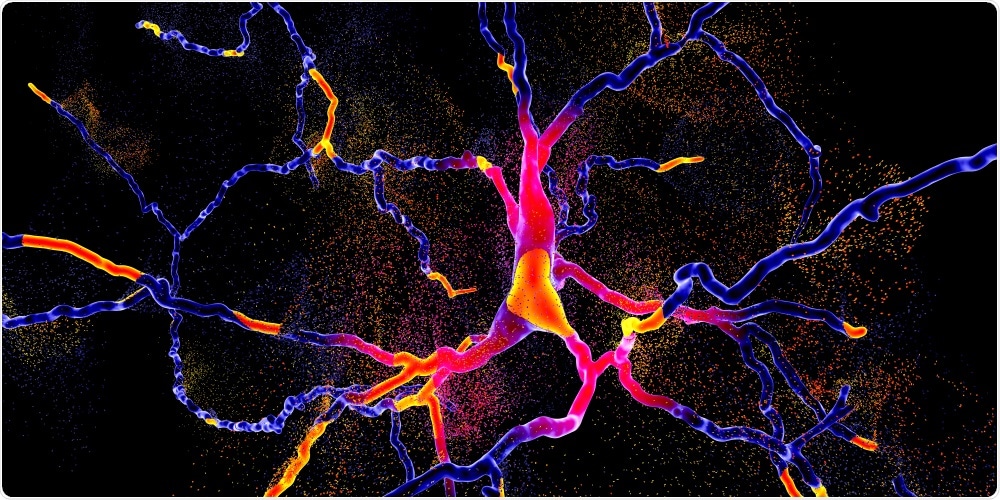
Image Credit: Kateryna Kon/Shutterstock.com
Why is it important to be able to accurately determine the chemical and structural properties of biomolecules?
Nowadays in society, there are several complex grand challenges to be solved, such as human diseases and neurodegeneration. It is generally understood that an interdisciplinary approach is central to solving them. When biosciences and nanotechnology started in the 20th-century people were very excited about being able to “see” biomolecules at the nanoscale.
However, this morphological information was not sufficient, and people realized that in order to understand how a biomolecule works in our brain or in our body, it is necessary to understand how its function is related to its structural and chemical properties, which define their physiological function.
Someone once said that proteins are actors in the theatre of the body, but in order for each actor to have the right role, its structure and chemical properties must be well defined.
What provoked your research into imaging techniques and biomolecules?
One of the major focuses of my research is unraveling the molecular basis of neurodegeneration exploiting single-molecule approaches. These diseases are related to a conformational change (misfolding) of the proteins in our brain (which have a certain structure and chemical properties). This change leads to an aggregation process, which is extremely heterogeneous, and this leads to the formation of several amyloid oligomeric and fibrillar species, which have transient, heterogeneous, and nanoscale nature.
It is not fully clear which amyloid species are involved in toxicity and involved in the molecular mechanisms causing neurodegeneration, thus there is the need to characterize these species one by one at the nanoscale to understand which one(s) could be the putative cause for toxicity. For this, you need imaging.
However, as said before, looking only at their morphology is not sufficient, it is also necessary to understand their chemical and structural properties at the single-molecule scale. This is why nanoscale chemical imaging and spectroscopy have been both useful and necessary for my research.
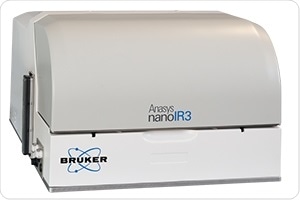
Image Credit: Bruker
How have spectroscopic methods advanced throughout the years, and what new discoveries has this brought?
Around five years ago we began exploring the application of infrared nanospectroscopy in biology using the nanoIR1 system (Bruker/Anasys Instruments) to demonstrate that it was able to study this heterogeneity in aggregating proteins. We demonstrated for the first time at the single aggregate scale the molecular structural changes that a protein undergoes during amyloid formation.
However, at the time, the technique was only able to measure large supramolecular aggregates with 100- or 200-nanometer minimal dimensions. Moreover, it was only able to acquire structural and chemical information in air environments.
Today, we have pushed forward the boundaries of nanoscale chemical imaging and spectroscopy down to the single-molecule at the nanoscale, still in an air environment. Recently though, we have also demonstrated that the technique is already capable of acquiring chemical information at the nanoscale in a liquid environment, which is a much more physiological environment for the study of biomolecular processes; thus, paving the way to open a new label-free window of observation in biology at the nanoscale and physiological conditions.
Obtaining vibrational absorption spectra at the single-molecule level for these biomolecules, however, has remained difficult. Why is this?
The tricky part of studying biomolecular processes is that, in most cases, they happen at the nanoscale. The biomolecules involved have nanoscale intrinsic dimensions, so they are very difficult to see with conventional microscopy methods. It is also difficult to study them with bulk spectroscopy methods because as well as having nanoscale dimensions, they are also heterogeneous.
Microspectroscopy has a limited spatial resolution, and in general, microscopy does not allow us to retrieve information on single-molecule species – it only provides information on a population’s average, which is not sufficient to unravel in detail biomolecular processes.
In recent years, there have been many nanoscale spectroscopic techniques that have tried to characterize the chemical properties of biological systems at the nanoscale. However, there is currently a trade-off between sensitivity and high spectra reliability and ease of interpretation.
On the base of this trade-off, we can divide current state-of-the-art methods into two categories: scattering methods, which can have rich extreme sensitivity, but often are limited by high spectral complexity and reproducibility, and which require complex modeling to retrieve chemical information; and on the other hand, thermomechanical methods, such as infrared absorption nanospectroscopy (AFM-IR), which measure directly and purely the IR absorption of the sample and assure high spectra reproducibility and interpretation.
However, until now, the sensitivity of infrared absorption nanospectroscopy was limited because there were difficulties in understanding the principles at the base of thermomechanical detection. Moreover, the technique uses a field enhancement at the metallic nanogap between a sharp tip and the substrates where the proteins are deposited but understanding how this field enhancement can affect the measurements has been difficult till now.
In your research, you introduced the imaging technique ORS-nanoIR. How could this imaging method help to obtain absorption spectra of biomolecules at the single-molecule level?
It was already known and reported in literature that using a metallic nanogap leads to an enhancement of the electromagnetic field, therefore improving the sensitivity of nanoscale chemical methods. Furthermore, as mentioned before, infrared nanospectroscopy is a thermomechanical-based method.
What does that mean if there is a protein on the surface? In this case, if we send IR light at the right frequency, it will be absorbed by the sample. This absorption causes a vibration of the chemical bonds, and this vibration is dissipated as heat, which causes a thermal expansion of the protein.
For a single protein, this thermal expansion is very small, and we use the tip of an atomic force microscope probe, which is in contact with the sample, to measure it. It can be demonstrated that the response of the probe measuring this expansion is proportional to the IR absorption.
There are two main factors limiting this method’s ability to reach the single-molecule scale. The first is being able to measure this expansion, and the second is being able to preserve (not destroy) the protein, because there is a very hard tip on the top of it.
To address these issues, we first started to look at how the field enhancement at the metallic nanogap between the protein and the surface can influence the signal-to-noise ratio of the measurement. Normally people would assume that having a higher-powered laser would result in a higher signal-to-noise ratio because you are adding more enhancement. But we discovered that having too much laser power would instead cause large oscillations of the tip in contact with the sample, ultimately leading to non-linearity and destroying the sample. By completely lowering the laser power, we aimed to avoid destroying this very soft protein and preserving the linearity of the signal.
Then, we also looked at how the mechanical resonances of the cantilever could be tuned to enhance this signal. It was already understood that if you tune your IR laser to the same frequency as the oscillation of the cantilever, it is possible to enhance the signal. We discovered that we needed to shift the frequency of measurement a bit lower in order to be more sensitive to the protein and less sensitive to the gold that is around it, therefore resulting in an enhancement of the field.
Can you describe the advantages of using ORS-nanoIR over other imaging methods commonly used, such as conventional vibrational spectroscopy? How sensitive is this imaging method?
Vibrational spectroscopy or microspectroscopy has a typical spatial resolution in the order of a few microns, while a single protein has dimensions in the order of one to ten nanometers. Conventional bulk vibrational microspectroscopy is not able to study a single protein, so I have developed Off-Resonance, Short Pulse, and Low Power Infrared Absorption Nanospectroscopy (ORS-nanoIR) to address this technological gap using the nanoIR2 system (Bruker/Anasys Instruments).
The method enabling us to accurately acquire chemical maps and spectra at the single protein molecule level. Then, the study of the shape and position of their Amide band I allow us to do very accurate characterization of their secondary structure. The main advantage of ORS-nanoIR and, in general, thermomechanical detection, is that the IR spectrum we acquire is a direct measurement of the absorbed light by the sample. This means that we are only and directly measuring the infrared absorption, without any scattering effect.
Secondary structure characterization is important in studying neurodegeneration. For example, Alzheimer's disease or Parkinson's onset is related to misfolding of proteins in the patients’ brain, causing aggregation and changes in their secondary structure. This change causes the loss of protein function or the sequestration of other biomolecules, which in turn then disrupts physiological behaviors and pathways.
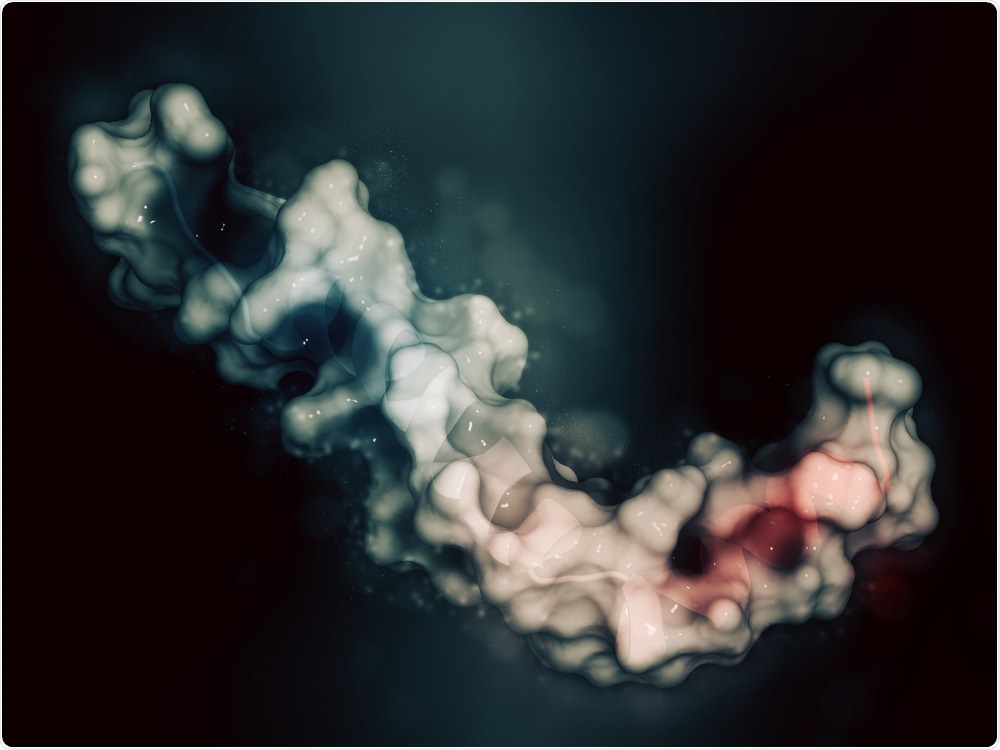
Image Credit: StudioMolekuul/Shutterstock.com
Do you believe that your results from ORS-nanoIR can help to better identify and characterize these biomolecules, which in turn, would help to understand more biochemical processes?
Biomolecular processes are based on the relationship between the structures, chemical state, and function of biomolecules and on how these states change during biomolecular interactions. If you think of the function of a cell, there are hundreds of biochemical processes regulated by lipid membranes and vesicles, proteins, and nucleic acids. It is necessary to understand how these processes happen at the single-molecule level.
ORS nano-IR will enable us to directly characterize these processes for the first time. Until now, no technique has been able to characterize chemical interaction at the single-molecule scale in such detail and with such high throughput. The ORS-nanoIR technique opens up a completely new field of observation in biology on the structural and chemical properties of biomolecules at the single-molecule nanoscale.
Where do you see the future of imaging methods heading? What further advancements could be made to further develop the sensitivity of this technique?
Current state-of-the-art technology has reached single-molecule sensitivity in air environments, and we are able to measure in liquid environments, but this is still at a resolution similar to techniques five years ago – in the order of tens of nanometers, rather than at a single-molecule scale.
A fundamental advance would be to successfully apply infrared nanospectroscopy in biosciences in order to reach single-molecule detection in a liquid environment. I plan on addressing this in my future research with the nanoIR3 Fluid AFM-IR system (Bruker).
What are the next steps in your research into nanoIR and biomolecules?
In my research program, I focus equally on both the technological development of infrared nanospectroscopy and applications in bioscience and biomaterials.
For example, I applied infrared nanospectroscopy for the characterization of innovative functional artificial biomembranes. Moreover, I recently demonstrated that infrared nanospectroscopy could successfully study a very interesting and novel biological phenomenon, which is the process of protein liquid-liquid phase separation.
Until a few years ago, it was thought that every organelle within a cell was surrounded by a lipid membrane, but now it is clear that there are organelles capable to solve their physiological function without the need for a lipid membrane and that assemble through liquid-liquid phase separation. One of the main objectives of my future research is to unravel the molecular mechanisms of liquid-liquid phase separation in physiological liquid environments with nanoscale chemical and structural resolution.
Furthermore, I plan on expanding my initial approach from in-vitro samples to ex-vivo human biopsies in order to identify protein aggregates in the brains of patients suffering from Alzheimer’s and Parkinson’s disease. We can then, for instance, use the spectroscopic chemical information that is contained in the nanoscale localized chemical spectra as biomarkers of disease.
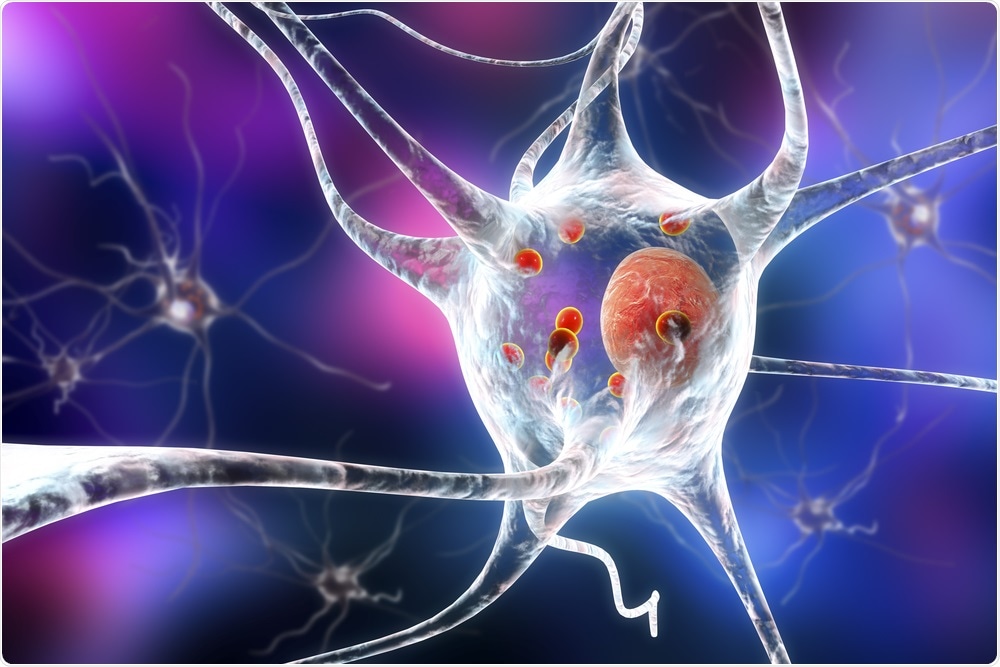
Image Credit: Kateryna Kon/Shutterstock.com
What are some things you know from your work that you wish more people knew?
For an external person looking at my research, which is focused on protein self-assembly and neurodegeneration, it may appear that our use of infrared nanospectroscopy is largely based on current applications with little development. However, demonstrating single-molecule sensitivity has already shown that in order to solve very complex problems, we need to advance our technology significantly.
This is not always clear, however, because our scientific outputs have mostly related to interdisciplinary work and are very applied. I would like more people to know that we now have to constantly expand the capabilities of atomic force microscopy and nanospectroscopy in order to solve fundamental problems in bioscience.
About Francesco Simone Ruggeri
I currently work at the Centre for Misfolding Diseases at the University of Cambridge. The focus of my original research programme is to develop and apply new Physical methods at the interface with Chemistry and Biology to shed light on the molecular processes underlying the onset of neurodegenerative disorders and study functional biomaterials for biomedical applications.
In my research, I continuously push the boundaries of the methods of analysis of modern biology and physics for investigating complex and heterogeneous biological samples and biomaterials at the nanoscale. I have deep expertise in scanning probe microscopy, surface science, spectroscopy, data analysis, image processing and single particle characterisation.