Multi-angle light scattering (MALS) is a powerful tool for directly measuring the molar masses of proteins, nucleic acids, and complexes in solution without fluorescent or radio-labeling.
In this interview, Dr. Sophia Kenrick talks to News-Medical Life Sciences about two complementary light-scattering techniques, size-exclusion chromatography coupled with multi-angle light scattering (MALS) and composition-gradient MALS that provide characterization tools for analyzing nucleic acids and the interactions between nucleic acids and proteins.
Can you explain the importance of studying protein-nucleic acid interactions?
The biotherapeutics being developed today for the treatment of numerous disorders are based on a wide range of biopolymers. Typically, biotherapeutics were engineered antibodies, but now all kinds of biopolymers are being used to produce therapeutic agents. DNA- and RNA-based biotherapeutics are becoming increasingly prevalent as cancer immunotherapies and cancer vaccines.
The efficacy of such treatments depends on RNA being delivered to immune cells and transforming them into tumor-targeting agents. The delivery aspect is crucial to its efficacy. It is therefore important that we understand the properties of the biopolymers being used and how they interact with other biological compounds in order to make them as effective as possible. Biophysical characterization is thus essential. For example, the delivery efficiency could be dependent on how much polymer is conjugated to your RNA or the purification process may cause your single-stranded RNA to form duplexes that you don't want.
Characterizing the interactions between nucleic acids and host proteins or other molecules is also critical to understanding the underlying biology of both healthy and disease states. Getting detailed information about how these molecules bind helps us understand structure/function relationships and informs the development of sensitive or high-affinity biosensors.
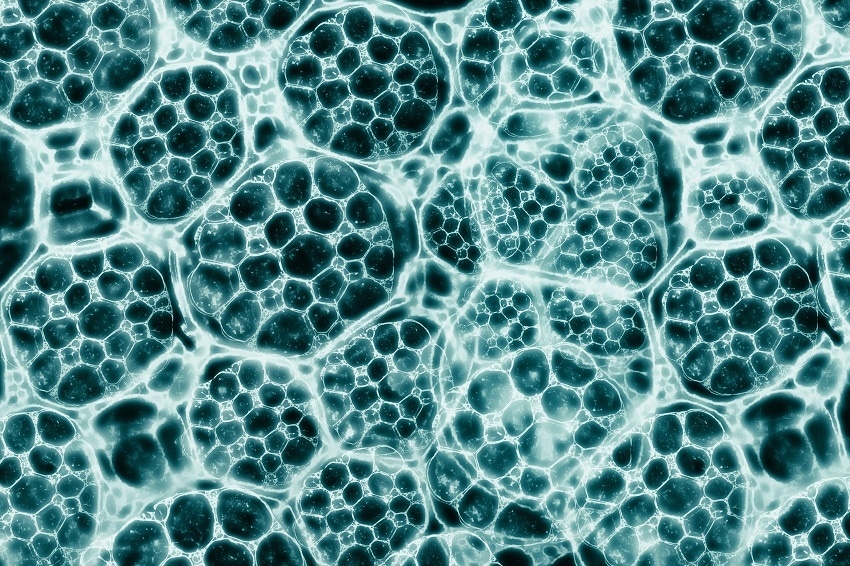
Image Credit:Shutterstock/GiroScience
How can light scattering be used to characterize such interactions?
Light scattering studies can provide key information about the size, molar mass, and conformation of these complexes. Both the molar mass of the molecules and the strength of the interactions with their binding ligands can be directly measured without the need for labeling or molar mass standards. This reduces the risk of artefacts.
There are several ways that light scattering techniques can be applied to DNA and RNA characterization. MALS, dynamic light scattering, and electrophoretic light scattering can all be used to characterize physical properties, such as size, molecular weight, and charge.
Which of the light scattering techniques were used in the case studies?
Both case studies use multi-angle light scattering or MALS. When a laser is directed at a solution, most of the light passes all the way through, but some of the light is scattered in all directions by the molecules that are in the solution. The intensity of the scattered light is proportional to the molar mass, the concentration, and the refractive index increment, or dn/dc. So, by measuring the light scattering intensity you can directly calculate the molar mass of those molecules in solution.
The light scattering is measured at multiple angles simultaneously. This is important as the variation in the scattering angle is proportional to the size of that molecule and can be used to determine the radius of a molecule or particle, and this is critical for understanding larger DNA and mRNA molecules.
The first case study shows the use of MALS with size exclusion chromatography (SEC-MALS) in characterizing the complex of DNA and protein by analyzing each of the species present in a solution. The second case study uses CG-MALS to directly measure the binding affinity and stoichiometry of the complex in solution.
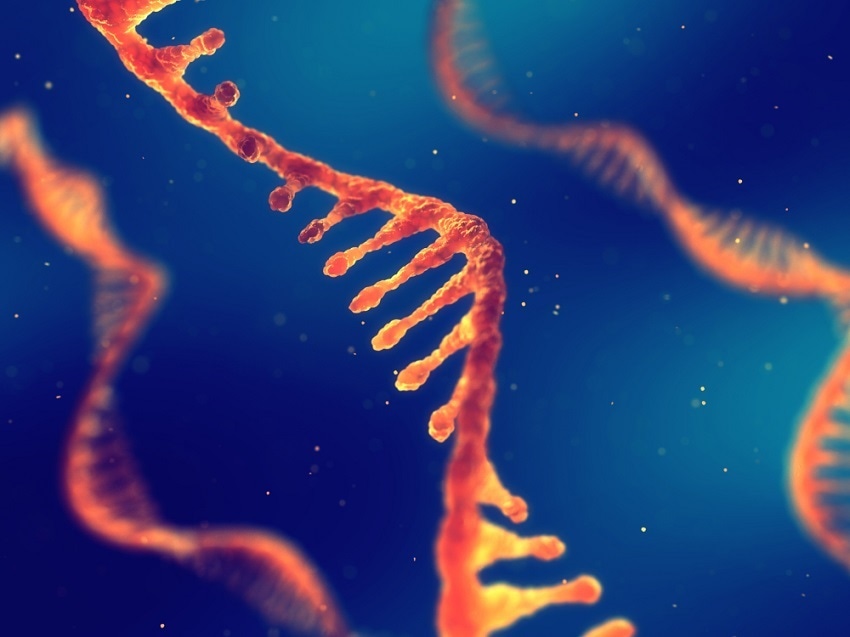
Image Credit:Shutterstock/nobeastofierce
How is SEC-MALS used in the study of biological complexes?
Sometimes, even when an HPLC or UHPLC separation is ideal, the molar mass of the sample estimated just based on the elution time does not match the molar mass of the standard. This mismatch can arise when the sample and the standard have a different density and a different conformation.
In SEC-MALS the effluent from an HPLC or UHPLC column flows into a UV detector, our multi-angle light scattering detector, and a refractive index (RI) detector. The UV or the RI detector provides the concentration measurement, which is used along with the MALS intensity data to calculate the true molar mass. The MALS measurement also confirms that the solution has been completely separated by revealing whether the peaks are uniform in terms of molar mass, or heterogeneous.
This analysis is not as simple for a complex consisting of two different types of molecules. Each component has a different extinction coefficient and a different dn/dc. In such cases, we need to apply a “protein conjugate analysis” that employs all three detectors determine the amount of each component in our complex, directly.
Measuring the concentration using both the UV and the RI detector, gives us the mass fraction and the molar mass of each component. There are multiple applications for this technique, such as glycosylated or pegylated proteins, or a polysaccharide functionalized with peptides or with nucleic acids. Even non-covalent complexes can be characterized, such as the amount of lipid associated with a membrane protein or, in our case protein-nucleic acid complexes.
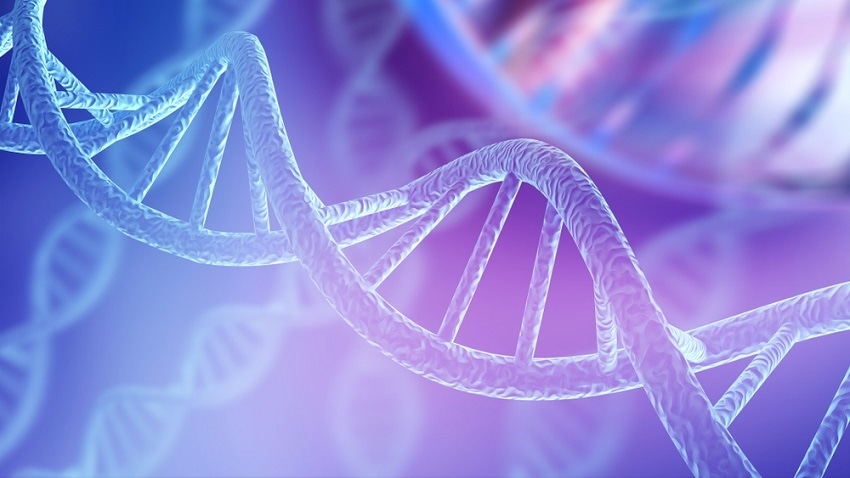
ImageCredit:Shutterstock/Billionphotos
How was this applied in your SEC-MALS case study?
The SEC-MALS and protein conjugate analysis approach has been applied to the complex of prototype foamy virus (PFV) integrase and viral DNA, which is called the intasome.
The extinction coefficient obtained for PFV integrase using UV and the RI simultaneously was almost half of what we would expect just based on the sequence, but measuring the molar mass both with the RI concentration and the UV concentration confirm that it is correct. Already, this is useful information about the complex that will help in determining the molar mass.
Moving on to the intasome, the light scattering, UV, and RI signals were used to calculate the molar mass and the mass fractions of each part at every point in the chromatogram.
This showed that the protein fraction is about 90%, meaning 90% of the mass is protein and about 10% is DNA. Based on this information, we got the dn/dc for the entire complex and the extinction coefficient for that entire complex. We used this concentration and this dn/dc to then calculate the molar mass. The total molar mass was 194 kilodaltons, so we know that the protein part is 174 kilodaltons, and the DNA part is about 20 kilodaltons. This is equivalent to a tetramer of the integrase and two DNA strands, which matches the crystal structure.
It was observed that the molar mass was not constant across the peak, indicating that dissociation of the protein tetramer may have occurred during a separation or some species may not be saturated. The next case study shows how we can measure these kinds of affinities and stoichiometry in solution.
So how does CG-MALS differ from SEC-MALS?
CG-MALS is the technique that lets us measure the affinity and the stoichiometry of complexes forming in solution.
Unlike in the previous case study, MALS is applied to unfractionated samples. A series of samples of different compositions are injected into the light scattering and concentration detectors so we can use MALS to determine the molar mass at each composition. The change in the molar mass can then be related to the formation of complexes in solution.
In this way, we can assess self-association and the equilibrium between monomer and dimer and dimer and trimer. By measuring the molar mass as a function of concentration, we can determine the self-association affinity and the stoichiometry.
If no complexes form, the molar mass will stay constant as a function of concentration. As complexes like dimers and trimers form, we see an increase in the molar mass as a function of concentration. Using an appropriate model, we can fit this increase to tell us the affinity and the stoichiometry of the association.
The same technique can be applied to an interaction between two different species. The composition just includes varying concentrations of both species. We get measurements when each of the species is in excess so we can sample the entire stoichiometry of that interaction.
As complexes form, the molar mass of that solution increases giving a characteristic curve. The more complexes that bind, the stronger the interaction. This makes the peak higher and sharper.
Also, the position of the peak tells us about the stoichiometry of the solution. If species B has one binding site for species A, we'll see the peak apex at the 1:1 molar ratio. If each B can bind two A molecules, we'll see the maximum at the 2:1 molar ratio and so on.
Also, since the CG-MAL technique measures molar mass directly, we can tell the difference, say, between a 1:1 and a 2:2 interaction. They'll both reach a maximum at the same position, but we can still differentiate as the 2:2 complex will have twice the molar mass.
Which complex was studied using CG-MALS in your second case study?
CG-MALS was used to investigate a Cre recombinase in complex with a loxP DNA recognition site.
Firstly, we used a concentration gradient of the Cre to measure the molar mass of that protein alone and determine if it self-associates. A similar test was conducted for the DNA by itself. MALS measurements were taken for each molecule at five different concentrations.
The molar mass of each molecule did not increase across the concentration range, showing that they're not self-associating at all. The Cre gave a constant molar mass of 39 kilodaltons, which is consistent with a monomer, and the DNA molar mass measured a constant 23 kilodalton, which is also the monomer.
The association between the enzyme and the DNA was then studied. Mixtures of the protein and the DNA at 13 different concentrations were analyzed in the MALS flow cell. The increase in the light scattering intensity was proportional to the increase in the molar mass over time, as the Cre/loxP complexes were formed.
The molar mass at equilibrium was found to be around 110 kilodaltons. This immediately told us that the stoichiometry is greater than 1:1 as 39 plus 23 is only 62.
The peak was in the right position for a 2:1 binding ratio, but the molar mass was still greater than it should be for such a complex, suggesting that some higher order structures are being formed rather than a standard 1:1 or 2:1 complex. This was supported by the curvature at the high ratios of DNA to a protein that is not being captured by a simple 2:1 model.
Measurement of the molar masses showed that there were actually three different complexes. The first one is a Cre protein bound to the DNA, and this first binding occurs with an equilibrium dissociation constant of 170 nanomolar.
A second Cre then binds to this complex in a cooperative manner. Once one Cre protein is bound to the DNA the affinity for the second one increases. Finally, this Cre-loxP hetero-tetramer dimerizes with an affinity of 400 nanomolar.
A single CG-MALS experiment exploring concentrations of just a few hundred nanomolar for each species thus enabled us to describe this three-step binding mechanism fully.
It wasn't necessary to work in multiple concentration regimes or assume that one or more of these binding sites is fully saturated, or that one part of this reaction is negligible. You can get complete characterization and measure all of it with just a single experiment.
Not only do you get the affinity and the stoichiometry, but CG-MALS also gives you the composition of the solution for each mixture.
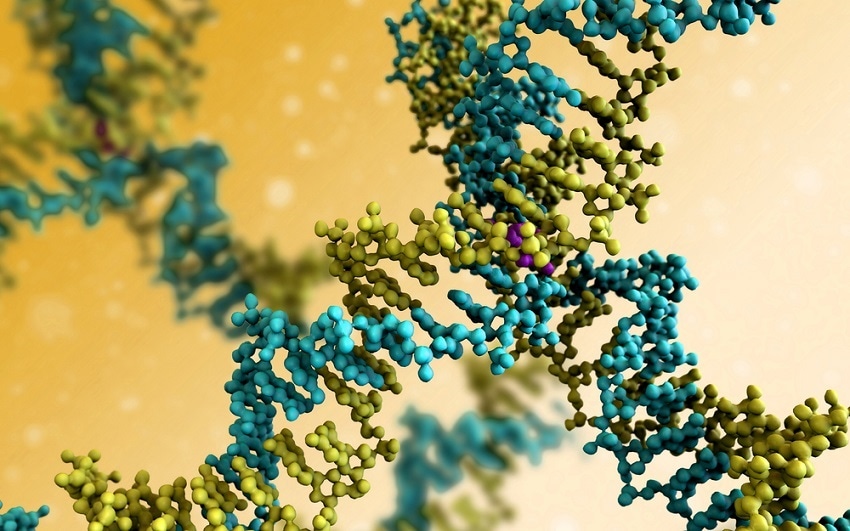
Image Credit:Shutterstock/UGREEN3S
Can you give us a brief summary of the two case studies?
Yes, hopefully, I've also shown that light scattering, either with fractionation or in batch mode, is not just for proteins. These same analyses are critical for DNA and RNA, and for characterizing nucleic acids in complex with other molecules.
The two case studies highlighted two different kinds of characterizations that can be obtained for similar protein/DNA systems using MALS.
SEC-MALS with protein conjugate analysis gave us the absolute molar mass and the protein and DNA fraction for a viral integrase bound to its DNA ligand. This was done without molar mass standards and without assuming anything about the stoichiometry.
CG-MALS in batch mode without chromatography lets us quantify the affinity and the stoichiometry of that complex in a multi-step protein/DNA interaction. The interaction analysis does not require labelling or immobilization.
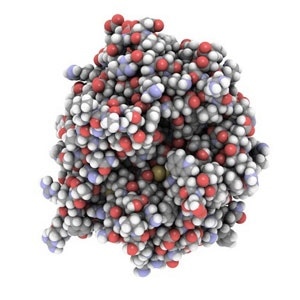
Where can our readers go to find out more?
To find out more, please visit our website - www.wyatt.com/SEC-MALS and www.wyatt.com/CG-MALS.
About Sophia Kenrick
.jpg)
Dr. Sophia Kenrick is a senior application scientist at Wyatt Technology where she supports multiple applications for Wyatt Instrumentation, especially in the field of molecular recognition and biomolecular interactions.
Sophia serves as the Director of Analytical Services and as the Dean of Light Scattering University, Santa Barbara Campus.