Introduction
This article is an executive summary of a webcast titled “Polymer and Processing Challenges for the Next Endovascular Revolution,” sponsored by Zeus and published on October 21st, 2015.
When lipids are deposited within the vessels, it leads to subsequent plaque formation along the vessel walls, in turn resulting in arterial disease. Such plaque lesions could remain soft or turn hard and calcified; over time, they could reduce the luminal space within the vessel due to the accumulation of plaque. Earlier, balloon angioplasty was used to treat this narrowing of the vessel interior, called stenosis. This process involves traversing a balloon across the vessel lesion and expanding it to push the narrowed vessel outward to restore the flow of blood. A subsequent enhancement to balloon angioplasty was the approach of bare metal stents (BMS), in which free dilatation was performed by inserting a second balloon loaded with the metallic stent following the first angioplasty balloon. Then, the metallic stent was expanded in place to broaden the narrowed artery and to restore normal blood flow.
However, continuous application of the BMS technology revealed various complications. Certain BMS implantations led to excessive formation of neointima both inside and around the stent as the body attempted to dispense the foreign item. The arterial lumen was narrowed by the consequent hyperplasia and overgrowth of the new intimal layer, thereby effectively reversing the effect of the BMS.
Hence, an innovative stent technology was devised to address hyperplasia-associated vessel blockages from BMS. Drug-eluting stents (DES) enable the release of anti-proliferative drugs from the stent once they are implanted. Such stents were developed to minimize the hyperplasia associated with BMS and prevent vessel blockages after implantation. In the consecutive years, efforts made in the medical industry to devise a technology that would realize a perfect combination of the potential of the stent to push open the artery and deliver the drugs as needed to regulate the response of the body to the foreign entities. In addition, a methodology for enabling the vessel to be restored back to a state closer to normal was sought after. This study enabled the development of bioresorbable vascular scaffold (BRS) technology, where scaffolds are implanted arterially and are taken up by the body over time as they finally disappear.
Various drawbacks of earlier stent therapies (Table 1) have been overcome by bioresorbable scaffolds. For treating acute occlusion caused by balloon angioplasty, metal stents were adopted as a standard treatment owing to their strength and ability to maintain luminal diameter. Apart from offering the same benefit, bioresorbable stents also prevent the associated hyperplasia and risk of constrictive remodeling. To date, it was not possible to deliver therapeutic agents during balloon angioplasty. Although DES offer this potential to address proliferation of new tissue, they also lead to consequences related to implanted foreign entities.
Even in this aspect, BRS technology is successful since the bioresorbable devices are taken up by the body, preventing hyperplasia in majority of the cases. BRS technology also enhanced the extent of remodeling after angioplasty or stent placement. Although balloon angioplasty, in general, demonstrates greater remodeling than BMS or DES, bioresorbable scaffolds support extensive remodeling since the implanted device is taken up. As the scaffold no longer exists, natural strain and stress as well as normal vasomotion in arterial wall motion are more exactly approximated, thereby stimulating natural remodeling of the arterial wall.
Table 1. Potential benefits of BRS (+ Prevented or not restricted; – not prevented or restricted; NA not applicable because of the absence of stent)
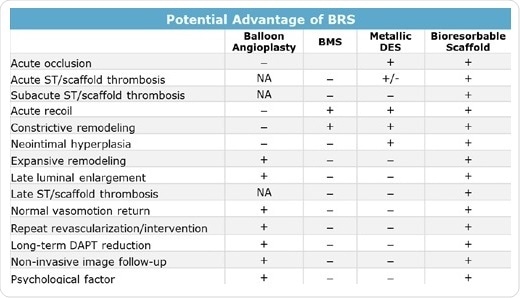
The Path to BRS
Percutaneous coronary intervention (PCI), or coronary angioplasty, is a specialized and tested method that particularly targets blocked coronary arteries. Undeniably, a large amount of historical clinical data supports the revolutions in PCI technology. Take for example a 15 year follow-up study after BMS implantation which demonstrated that the cumulative target lesion failure (TLF) at five years was ~23%, and that at 15 years, the lesion failure rate was ~50% (Figure 1A). In another similar study, cumulative TLF rates for two DES were compared (Figure 1B). In this study, it was found that for a paclitaxel-eluting stent (PES) called Taxus, from Boston Scientific, TLF after five years was ~19%. For an everolimus-eluting stent (EES) called Xience, from Abbott, TLF was ~13%, which is now regarded to be the best-in-class.
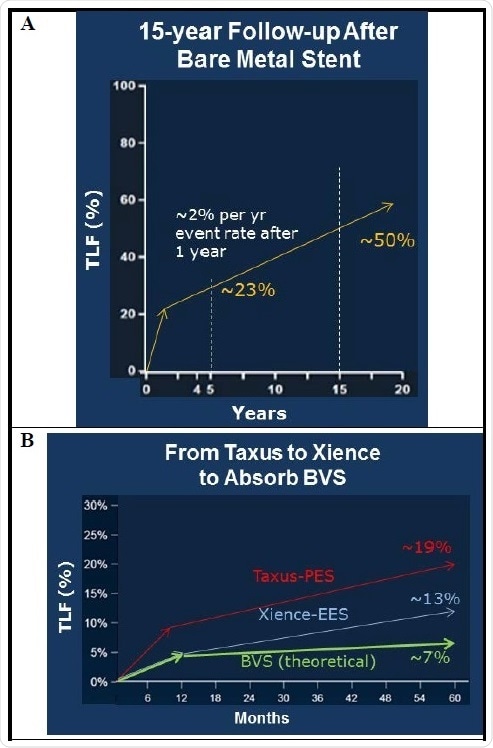
Figure 1. Clinical data showing the development path from bare metal stents to bioresorbable scaffolds. Cumulative total lesion failure (TLF) includes cardiac death, target vessel myocardial infarction, ischemia-driven target lesion revascularization.
When compared to Taxus and Xience DES, a successful BRS should initially demonstrate commensurate clinical efficacy but exhibit significant improvements as time passes (Figure 1B). Once the BRS is implanted, it is anticipated to demonstrate equivalent best-in-class TLF results as against stents such as Xience for approximately a year. Following one to five years after being implanted, BRS is anticipated to exhibit an evident decrease in TLF than other DES as the BRS is taken up by the body and arterial physiological function reaches normal. In case these expectations of BRS are satisfied, BRS will turn out to be the next major innovation in endovascular therapy.
Besides these long-term studies, a number of generations of BRS have been clinically tested for many years. One-year results of the progressive Absorb III (Abbott) bioresorbable vascular stent (BVS) clinical trial were published in 2015. In this prospective study, which is a multicenter single-blind trial, 2000 patients were given either a 2:1 ratio of Absorb BVS or Xience CoCr-EES. Plans for a five-year follow-up were included in this study. The Absorb program’s aim is to present a non-inferior result with Absorb BVS as against Xience EES at one year and to present superior results of the Absorb BVS from one to five years. The results from this study demonstrated that at 12 months, Absorb BVS was statistically non-inferior to Xience for TLF.
In spite of achieving non-inferiority for the Absorb BVS, broader results favored the Xience EES. A major concern was the fact that there was a higher level of scaffold thrombosis in the Absorb BVS study arm, specifically for stents implanted in smaller arteries. It was considered that the increase in scaffold thrombosis was due to the combination of the thick (157 μm) strut along with unsuitable or poor implantation techniques. While using a thick strut scaffold, it is advisable to use proper implantation methods: In all cases, post-dilation with a high-pressure non-compliant balloon is suggested — a practice which is absolutely different from the prevalent DES method. Post-dilation guarantees complete expansion of the scaffold to realize effective strut apposition. It is possible to enhance the overall method by embedding the strut(s) into the wall or plaque, thereby effectively reducing the strut thickness.
Why Is Strut Thickness Important?
Besides the probability of restenosis related to stent implantation, this treatment procedure also has the possible risk of thrombosis. Although the risk level is low, it poses a grave threat after stent implantation. Strut thickness is a factor that has been related to the rate of stent thrombosis. After implantation, a region of low endothelial stress occurs due to a large area of recirculation on the distal side of the stent strut. This low-stress region favors the aggregation of platelets, thereby resulting in thrombosis. Such recirculation can be minimized by using thinner stent struts, which help maintain physiologic endothelial shear stress to be close to homeostatic. Consequently, the aim of the advanced BRS should be to achieve considerably thinner stent struts.
The Ideal BRS
Achieving a perfect BRS is a multi-step procedure and mandates careful considerations. Of these considerations, the most consequential is the bioabsorbable tubing from which the BRS is developed. Preferably, the tubing should have low leachables, thermal stability, strength, strength retention, toughness, and the potential to curb the degradation of the material (Table 2). Thus, these physical characteristics of the tubing have an ultimate effect on the features of the BRS itself.
Table 2. Table showing the relationship of bioabsorbable stent attributes, bioabsorbable material properties, and the stent tubing specification targets.
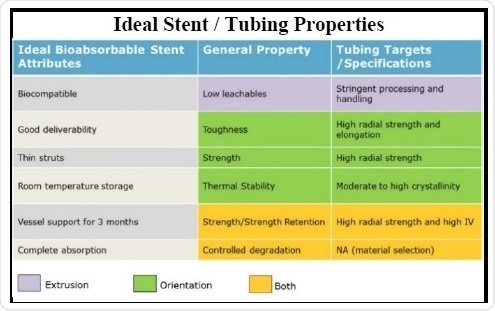
In relation to the practical aspects of the BRS, various desirable traits have been unearthed. Thus far, the most significant among these is biocompatibility, which is primarily realized by using the standard class of bioabsorbable polymers, mainly polyesters. These materials have a long-known record of proven biocompatibility in various medical devices; they also have well-controlled, tangible polymer handling and extrusion processes to inhibit contamination and to reduce polymer degradation. One more highly significant aspect is the good deliverability of the device. The scaffold should be able to withstand nominal expansion and over-expansion inside the vessel and maintain strut apposition. This quality can be realized by improving the toughness of the tubing using a combination of high radial strength and elongation resulting from optimal molecular orientation.
Scaffold strut thickness can be minimized by achieving high radial strength in the tubing. For increasing the service life and room temperature storage, the polymer can be processed to realize a moderate to high crystallinity. In the case of vessel support, the expert opinion is that scaffold or stent support should be continued for three months. This time frame is required for proper healing and remodeling of the stent site. Strength retention is mainly adjusted through the selected base polymer and subsequently by preserving a high molecular weight or viscosity over all the processing steps, thereby achieving a tubing with high radial strength.
Selection of polymer and molecular weight also enables the complete absorption timeline of the BRS to be controlled. The use polymers instead of metals poses its own set of problems. Intrinsically, polymers have lower toughness and strength when compared to many of the metals. This contrast from metal stents has to be carefully taken into account during scaffold designing, strut designing, and polymer processing. Optimal device design, for instance, could be undermined by poor polymer processing and vice versa. Taken together, the desirable stent material characteristics and stent features discussed here regulate the specifications required for the bioabsorbable stent tubing.
Processing Challenges for BRS
A complete knowledge of materials science and polymer chemistry is not adequate to achieve a clinically viable BRS. Development of these devices also mandates an in-depth understanding of the processing and handling related to the polymers. For instance, although the polymer chemistry plays a vital role in optimizing the rate of loss of mechanical integrity, processing at the time of the production steps also has a significant effect. Deficiencies in processing or handling of the polymer resins could result in compromised mechanical characteristics and absorption profiles of the tubing, which may cause undue inflammatory response and premature loss of mechanical support.
Tubing Manufacture
In the bioabsorbable tubing manufacturing technique, polymer chemistry with regards to morphology is also considered. For example, considering polymer selection, an ideal chemistry would include more of poly-L-lactic acid (PLLA) due to its higher glass transition temperature (Tg) and its potential to crystallize and lock in the molecular and crystallite orientation. The absorption profile of PLLA was also found to be consistent with the three-month critical mechanical support time limit. High-lactide copolymers — for instance, poly(L-lactide-co-ε-caprolactone) (PLC), poly(lactide-co-glycolide) (PLGA), and poly(L-lactide-co-D-lactide) (PLDLA) — are other polymers appropriate for bioabsorbable tubing. Co-monomer chemistry makes it possible to adjust the properties such as strength retention, toughness, and complete absorption profiles. Thus, cautious choice of polymer types and processing to form a particular morphology proves to be an added means to improve the overall performance of the BRS.
Besides the intrinsic properties related to polymer chemistry, Zeus has refined proprietary processes for producing one of the most sophisticated BRS device components to date. The high-precision and tight tolerance extrusion techniques developed by Zeus enables the focus to be on particular properties of bioabsorbable scaffolds. These processes involve management of the thermal, mechanical, and hydrolytic degradation of the polymer at the time of the handling and processing stages. This strategy also allows a highly perfected morphology development in successive processing stages. Therefore, careful consideration and foresight enable the use of these specialized processing methods to achieve ideal BRS characteristics for every application.
Orientation processing is a final step in the manufacture of tubing, which is carried out to direct the molecular arrangement of the polymer molecules in space to produce required material characteristics. For instance, this commonly adopted process is specifically popular with textiles and sutures, where the ensuing material orientation is in the machine direction. However, with respect to an annular geometry, there are additional considerations and opportunities to achieve more directed orientation. Directional orientation considerably enhances the toughness and radial tensile strength of the tubing (Figure 2). This processing step offers an additional mechanism to customize the properties of the BRS outside of any chemical considerations.
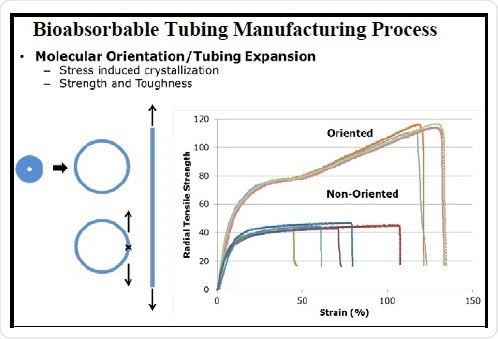
Figure 2. Illustration of the bioabsorbable tubing manufacturing process and the relationship of molecular orientation to strength and toughness.
Summary
In comparison with older generation stents, bioabsorbable scaffolds offer various important advantages. Such scaffolds portray a fourth revolution in the history of stent development and are becoming more popular with each new product that hits the marketplace. Their efficacy and safety at 12 months post-implantation and stabilized acute coronary syndrome were demonstrated by the Absorb III BVS trials. Moreover, thick struts of the first-generation bioabsorbable scaffolds emphasized the significance of post-procedural sizing and implantation process.
As a consequence, the focus of future-generation devices will be on using thinner struts to tackle these concerns and to reduce the risk of thrombosis and other detrimental events. Zeus employs scientists and engineers with a sound knowledge of morphology development, processing techniques, and bioabsorbable polymer chemistry. Thus, the company has now come up with a 100 µm wall bioabsorbable tubing particularly designed for BRS with optimal patient outcome, better expandability, and high strength.
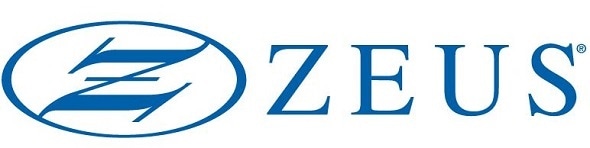
This information has been sourced, reviewed and adapted from materials provided by Zeus Inc.
For more information on this source, please visit Zeus Inc.