Please can you tell us a bit about Photon’s etc preclinical infrared imagers? What sets them apart from others on the market?
Photon Etc.’s (IR VIVO™) system is the first and only turnkey hyperspectral preclinical imager optimized for imaging in the second biological window of the infrared (NIR-II) / short-wavelength infrared (SWIR) range available on the market to our knowledge.
Our product portfolio includes different components of this preclinical imager, such as a hyperspectral filter (Hypercube™), widefield infrared imagers (S-EOS & GRAND-EOS) and cameras (ZephIR™ 1.7). This gives us a high level of control on the preclinical system, which may be customized to fit customer requirements.
Our standard IR VIVO™ preclinical system offers laser or LED illumination at wavelengths such as 730 nm or 808 nm, which are popular wavelengths for excitation of NIR-II markers such as carbon nanotubes. For detection, we can offer multispectral imaging using a filter wheel and hyperspectral imaging with a continuously tunable spectral range from 850 nm to 1620 nm and a spectral resolution of < 4 nm.
The system is also equipped with a heating plate, gas anesthesia inlet and outlet ports for up to 3 mice. Optional extensions include detection in the visible, which means that one could perform routine experiments carried out by standard preclinical optical imagers in addition to having the capability of doing hyperspectral and NIR-II imaging.
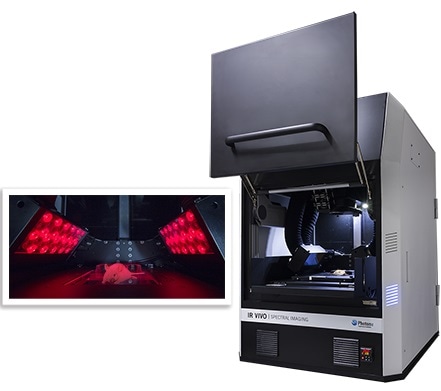
How does imaging in the second biological window differ from other optical imaging wavelengths?
There is reduced scattering as well as minimal absorption and auto-fluorescence by tissue when imaging in the second biological window (1000 to 1700 nm). As a result, there is a much better image contrast, sensitivity and penetration depth into tissue at these wavelengths than traditional visible or infrared optical imaging (i.e. 400-1000 nm).
Reaching a penetration depth of up to 3 centimeters has a huge impact when imaging small animal like mice, since it allows the visualization of full organs as well as cellular processes in real time with a high spatial resolution.
This positions NIR-II advantageously compared with other small animal imaging modalities. Indeed, MRI, PET and CT imaging can take up minutes to hours to complete a scan, are complex, costly and ionizing in the case of PET and CT.
Furthermore, they cannot provide the micron-scale resolution of NIR-II imaging. Finally, although the increased penetration depth in the second biological window does not allow imaging through the whole human body like MRI, PET or CT, its penetration depth is ideal for applications such as small animal imaging, surgical guidance or tumor resection.
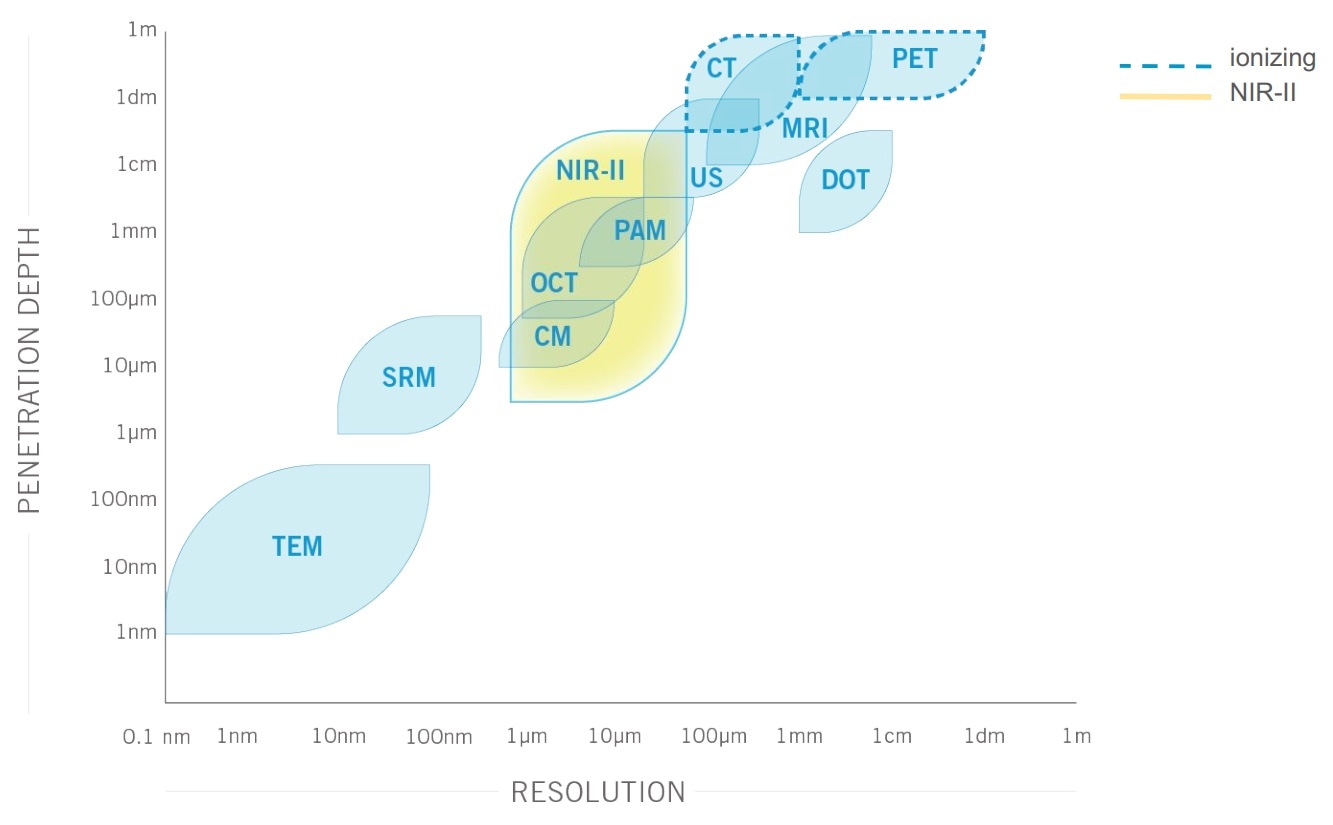
What is available in terms of technology at these wavelengths?
There are many infrared detectors available on the market. For imaging in the second biological window, indium gallium arsenide (InGaAs) based cameras offer the highest sensitivity. Originally restricted to the aerospace and military domain, InGaAs cameras are becoming more and more available to researchers.
Photon Etc. packages its own highly sensitive ZephIR™ 1.7 InGaAs camera operating at -80°C thanks to the integration of a four-stage TE cooler. This low operating temperature allows signal detection at extremely low noise levels.
As short-wave infrared (SWIR) cameras became more accessible, researchers began testing the potential of working at these wavelengths for life science imaging. They found that a better penetration depth and image clarity could be achieved with this technology, and started developing biomedical markers that could be used at corresponding wavelengths. These fluorescent markers are essential to allow targeting biological entities.
A few NIR-II markers are already commercially available. Although it has been traditionally used for imaging around 800 nm, clinical dye indocyanine green (ICG) turns out to also have a good emission around 1300 nm. Part of the strength of NIR-II imaging is that it unlocks the potential of NIR dyes which have long emission tails in the NIR-II by proving the much-needed resolution for biodistribution studies.
Promising markers which are currently being developed include small molecules, quantum dots, single-walled carbon nanotubes (SWCNT) and rare earth nanoparticles. Early results indicate that many NIR dyes have long emission tails. An extra imaging window will now greatly benefit research programs already in place that aim at making fluorescent dyes more targeted; the increased resolution that NIR-II optical imaging provides will further help translational research programs with their probe development.
Photon Etc. works hand in hand with researchers who develop these markers to provide them with imaging tools required for their development.
We offer one of the few microscopes optimized for infrared imaging available thus far on the market, the IMA™. Our microscopes offer the option to do infrared hyperspectral imaging, which allow rapid characterization of the emission wavelength of multiple markers.
Our preclinical NIR-II imager, IR VIVO™, was also developed to fulfill the demand of researchers working in the second biological window.
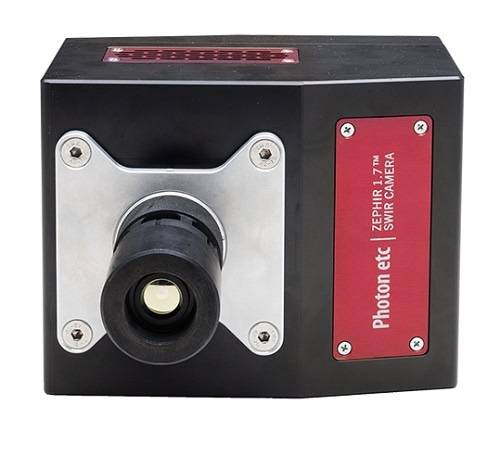
Caption: Photon etc.’s ZephIR 1.7, SWIR camera
Can you tell us about some of the sample applications of NIR-II Imaging?
NIR-II imaging is ideal for the rapid visualization of deep, small anatomical features with a high degree of clarity. Sample applications include blood flow, lymphatic or metabolic imaging. Tumor detection or cell environment assessment (lipid or microRNA content, pH, temperature, etc.) are other examples which will be discussed shortly.
Diseases related to blood flow such as peripheral arterial disease affect millions of people in America. Mouse models of ischemia are used to develop therapies to improve blood flow recovery. Studies have shown that NIR-II imaging can be used to monitor quantitatively microvascularization-related processes such as tissue perfusion, heart rate or blood flow in small animals.
Indeed, NIR-II allows both visualization of small microvessels at a spatial resolution higher than μCT imaging, and blood flow quantification at a speed matching ultrasound. These capabilities are also relevant for functional imaging of activity states such as muscle motion or brain response to stimuli, which are closely linked to perfusion.
A wide range of NIR-II markers or sensors are being developed to take advantage of the high penetration depth at these wavelengths. Examples include carbon nanotube sensors that allow detection of lipid or microRNA content. Depending on the cellular environment, the emission wavelength of the carbon nanotubes will be shifted and thus allow mapping of the fat content in real time.
It has been shown that changes in a mouse diet reflects lipid content changes. It is expected for this to provide a better insight in the detection and treatment of fat liver disease. In the same way, microRNA content can be assessed through wavelength shifts. In many cases, specific microRNA expression patterns are good disease indicators.
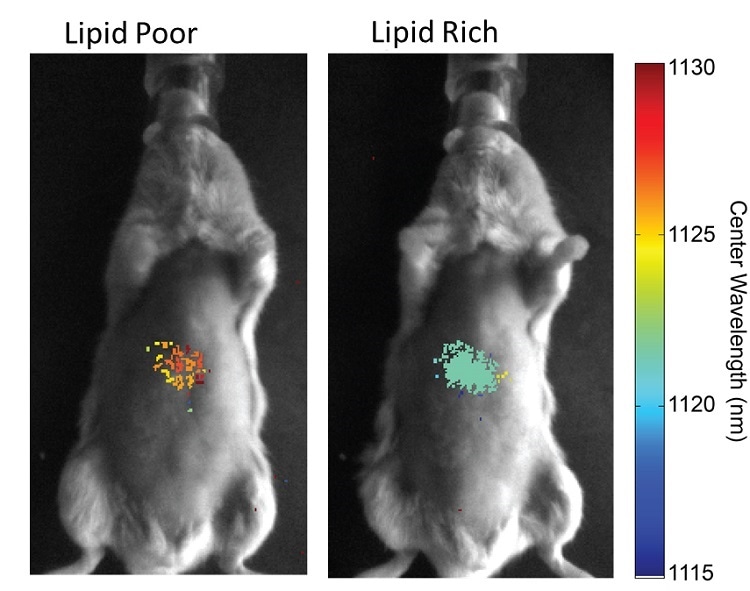
Source: Galassi, T. V., Jena, P. V., Shah, J., Ao, G., Molitor, E., Bram, Y., … Heller, D. A. (2018). An optical nanoreporter of endolysosomal lipid accumulation reveals enduring effects of diet on hepatic macrophages in vivo. Science Translational Medicine, 10(461), 1–10. https://doi.org/10.1126/scitranslmed.aar2680
Can the NIR-II imager identify cancer? What impact will this have?
NIR-II imaging can allow cancer detection thanks to intrinsic tissue properties or by using targeted markers. The increased penetration depth and contrast compared with visible imaging could allow superior tumor margin delineation or assessment of tumor volume.
In terms of tumor imaging metrics, it has been shown that using NIR-II markers can yield a significant improvement in tumor-to-normal (T/NT) ratio thanks to reduced background autofluorescence and scattering of tissue at these wavelengths. This means improved ability to detect tumors at earlier stages.
For research purposes, the detection of tumors at earlier stages and the monitoring of their progression may enable a better understanding of tumorigenesis and facilitate the assessment of treatment efficacy. NIR-II also enables monitoring the vascular activity, which is closely related to tumor growth through angiogenesis.
Clinically, NIR-II imaging has the potential to become a highly sensitive, rapid and inexpensive tool for intraoperative image-guided tumor resection. With the appropriate markers developed to target cancer, NIR-II imaging could reduce the incidence of positive surgical margins and therefore the risk of cancer recurrence.
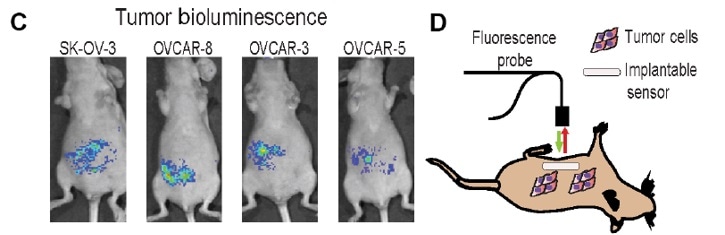
Source: Williams, R. M., Lee, C., Galassi, T. V, Harvey, J. D., Leicher, R., Sirenko, M., … Heller, D. A. (2018). Noninvasive ovarian cancer biomarker detection via an optical nanosensor implant. Science Advances, 4(4). https://doi.org/10.1126/sciadv.aaq1090
What does NIR-II imaging mean for the future of preclinical imaging?
NIR-II imaging will bring an unprecedented combination of fast, high resolution and penetration depth imaging at lower cost and to a broader community than current preclinical imaging techniques.
This will enable to resolve and track single biomedical targets or processes throughout small animals, thereby opening a new window of possibilities for fundamental and biopharmaceutical research.
Real-time biodistribution and pharmacokinetics of ICG in a mouse using NIR-II fluorescence.
Where can our readers go to find out more?
Please see our website page for IR VIVO™ system and IMA™.
Jena, P. V., Roxbury, D., Galassi, T. V., Akkari, L., Horoszko, C. P., Iaea, D. B., … Heller, D. A. (2017). A Carbon Nanotube Optical Reporter Maps Endolysosomal Lipid Flux. ACS Nano, 11(11), 10689–10703. https://doi.org/10.1021/acsnano.7b04743
Williams, R. M., Lee, C., Galassi, T. V, Harvey, J. D., Leicher, R., Sirenko, M., … Heller, D. A. (2018). Noninvasive ovarian cancer biomarker detection via an optical nanosensor implant. Science Advances, 4(4). https://doi.org/10.1126/sciadv.aaq1090
An optical nanoreporter of endolysosomal lipid accumulation reveals enduring effects of diet on hepatic macrophages in vivo
An in Vivo Nanosensor Measures Compartmental Doxorubicin Exposure
About Émilie Beaulieu Ouellet
.jpg)
A biomedical engineer by training, Émilie Beaulieu Ouellet is Application Scientist for life science imaging at Photon Etc. During her graduate studies at Polytechnique Montreal, she specialized in biophotonics, more specifically in the design of microscopes and endoscopes.
Émilie then worked as a biosystems field support specialist and technical representative for microscopy systems at Nikon Instruments. She later joined the Tearney group at Harvard Medical School as an optical engineer for the design and manufacturing of endoscopes for gastrointestinal imaging.
With 10 years experience in biophotonics, plus extensive exposure to varied applications in life science, Émilie strives to bring the latest innovations in biophotonics to the life science market.
REFERENCES
(i) Dion-Bertrand, L.-I. (2016). Hyperspectral Microscopy: A Powerful Technique for Multiplexed Imaging. Biophotonics, 23(8), 20–22.
Antaris, A. L., Chen, H., Cheng, K., Sun, Y., Hong, G., Qu, C., … Dai, H. (2016). A small-molecule dye for NIR-II imaging. Nature Materials, 15(2), 235–242. https://doi.org/10.1038/nmat4476
Ding, F., Zhan, Y., Lu, X., & Sun, Y. (2018). Recent advances in near-infrared II fluorophores for multifunctional biomedical imaging. Chemical Science, 9(19), 4370–4380. https://doi.org/10.1039/c8sc01153b
Bardhan, N. M., Dang, X., Qi, J., & Belcher, A. M. (2018). US 2018/0042483 A1. United States.
Dion-Bertrand, L.-I. (2016). Hyperspectral Microscopy: A Powerful Technique for Multiplexed Imaging. Biophotonics, 23(8), 20–22.
Lessard, Simon & Dion-Bertrand, Laura-Isabelle. (2017). COOLING SWIR SENSORS - AN OVERVIEW. 10.13140/RG.2.2.31365.65764.
Jena, P. V., Roxbury, D., Galassi, T. V., Akkari, L., Horoszko, C. P., Iaea, D. B., … Heller, D. A. (2017). A Carbon Nanotube Optical Reporter Maps Endolysosomal Lipid Flux. ACS Nano, 11(11), 10689–10703. https://doi.org/10.1021/acsnano.7b04743
Bruns, O. T., Bischof, T. S., Harris, D. K., Franke, D., Shi, Y., Riedemann, L., … Bawendi, M. G. (2017). Next-generation in vivo optical imaging with short-wave infrared quantum dots. Nature Biomedical Engineering, 1(4). https://doi.org/10.1038/s41551-017-0056
Hong, G., Lee, J. C., Jha, A., Diao, S., Nakayama, K. H., Hou, L., … Huang, N. F. (2014). Near-Infrared II Fluorescence for Imaging Hindlimb Vessel Regeneration with Dynamic Tissue Perfusion Measurement Guosong. Circ Cardiovasc Imaging, 7(3), 517–525. https://doi.org/10.1161/CIRCIMAGING.113.000305
Franke, D., Harris, D. K., Chen, O., Bruns, O. T., Carr, J. A., Wilson, M. W. B., & Bawendi, M. G. (2016). Continuous injection synthesis of indium arsenide quantum dots emissive in the short-wavelength infrared. Nature Communications, 7, 1–9. https://doi.org/10.1038/ncomms12749
Ghosh, D., Bagley, A. F., Na, Y. J., Birrer, M. J., Bhatia, S. N., & Belcher, A. M. (2014). Deep, noninvasive imaging and surgical guidance of submillimeter tumors using targeted M13-stabilized single-walled carbon nanotubes. Proceedings of the National Academy of Sciences, 111(38), 13948–13953. https://doi.org/10.1073/pnas.1400821111
Antaris, A. L., Chen, H., Cheng, K., Sun, Y., Hong, G., Qu, C., … Dai, H. (2016). A small-molecule dye for NIR-II imaging. Nature Materials, 15(2), 235–242. https://doi.org/10.1038/nmat4476
Galassi, T. V., Jena, P. V., Shah, J., Ao, G., Molitor, E., Bram, Y., … Heller, D. A. (2018). An optical nanoreporter of endolysosomal lipid accumulation reveals enduring effects of diet on hepatic macrophages in vivo. Science Translational Medicine, 10(461), 1–10. https://doi.org/10.1126/scitranslmed.aar2680