Sponsored Content by AbcamApr 18 2018
Proteins of the Hedgehog (Hh) family act as morphogens during development in vertebrate and invertebrate species. These powerful signaling molecules were initially discovered in a genetic screen conducted on a cuticle embryo to better comprehend the body segmentation of Drosophila melanogaster (Nusslein-Volhard and Wieschaus, 1980). In this genetic screen, mutant embryos for Hh developed as tiny prickly balls which were akin to a hedgehog (hence the name of the protein).
First identified in Drosophila melanogaster, the main components of the Hh pathway are preserved in vertebrates, where the pathway has retained the same mechanisms of action via species, albeit with some exceptions. Interestingly, developmental defects and cancer are caused by deregulation of the Hh pathway.
Hh signaling cascade in Drosophila
Hh maturation, release, and movement
Initially synthesized as a precursor, Hh goes through autoproteolytic cleavage where a palmitic acid molecule (Ingham and McMahon, 2001) and a cholesterol molecule (Porter et al., 1996) are added to the end product.
These modifications play an important role by directing the mature signal to communicate with a series of cellular components responsible for secretion, movement, and reception of Hh. Cholesterol, in particular, is involved in the trafficking and movement of Hh (Gallet et al., 2003), while palmitoylation plays a role in Hh signaling (Chamoun et al., 2001; Liu et al., 2007).
Once modified, Hh is ready to be released from the cells (Burke et al., 1999). Following its secretion, Hh easily interacts with the extracellular matrix and navigates through it in order to reach the receiving cells, where it eventually forms a concentration gradient.
A number of models have been suggested to demonstrate the way Hh moves away from its source; its movement within lipoprotein particles which are unique structures (Bolanos-Garcia and Miguel, 2003; Olofsson et al., 1999), and via its interaction with heparan sulphate proteoglycans (HSPGs) (Jia et al., 2003; Nakato et al., 1995), for example.
At the plasma membrane
At the plasma membrane, Hh signal transduction is initiated where Hh communicates with its 12 transmembrane protein receptor Patched (Ptc) (Ingham and McMahon, 2001). The Ihog/Cdo family of coreceptors facilitates Hh-Ptc interaction (Zhang et al., 2010). The Hh-Ptc binding has two major roles:
- Limiting the spreading of Hh: Internalization of Ptc and Hh occurs as result of their binding, targeting Hh to lysosomes for the purpose of degradation (Gallet and Therond, 2005).
- Increase of Smoothened (Smo) expression and activation: (Chen and Struhl, 1996; Denef et al., 2000; Lum et al., 2003; Taipale et al., 2002) this leads to a cascade of signal transmission that plays a role in the regulation of transcription factor Cubitus interruputs (Ci) (Alexandre et al., 1996; Méthot and Basler, 1999).
Following the binding between Ptc and Hh, the seven-pass transmembrane protein Smo goes through a number of phosphorylation events (Hh dose-dependent) (Fan et al., 2012). Phosphorylation of Smo takes place at its cytoplasmic tail (C-tail) that contains a number of phosphorylation sites of GSK3, PKA, and CK1(Zhang et al., 2004). Following are the key results of Smo phosphorylation:
- Inhibiting ubiquitation-mediated endocytosis and degradation (Fan et al., 2012) to promote the expression of Smo cell surface.
- Regulating Smo conformation, which takes place on the Smo dimer’s C-tail that results in an ACTIVE (C-tails opening and approach in the presence of Hh) and INACTIVE (C-tails distant from each other in the absence of Hh). Phosphorylation events contribute to this conformation change (Zhao et al., 2007).
Within the cytoplasm
A multi-protein complex (Hh signaling complex, HSC) downstream of Smo regulates the inhibition or activation of the Hh pathway. The components of the HSC complex are as follows:
- The serine/threonine kinase Fused (Fu)
- The transcription factor Ci
- Suppressor of fused (Sufu)
- Costal 2 (Cos2) is a kinesin-like molecule which also adheres to GSK3, PKA, and CK1, and is also implicated in the Hh signaling pathway (Aza-Blanc et al., 1997).
In the absence of Hh (Robbins et al., 1997; Sisson et al., 1997; Stegman et al., 2000), the HSC complex is associated with microtubules but in the presence of Hh it dissociates from the microtubule. The Cos-Fu-Ci complex then interacts with the Smo’s C-tail (Hooper, 2003; Ingham et al., 1991; Lum et al., 2003; Ogden et al., 2003; Ruel et al., 2003), while the Sufu-Ci complex stays cytoplasmic.
The status of the transcription factor Ci is regulated by both Sufu-Ci and Cos-Fu-Ci complexes. Being a 155 kDa protein (Ci-FL, full length), Ci contains a zinc finger domain which accounts for its DNA binding (Slusarski et al., 1995). Ci is transformed to an ACTIVE FORM (Ci-A, 155 kDa) which is responsible for activating a target gene in the presence of Hh, or it is converted to a REPRESSOR FORM (Ci-R, 75 kDa) that stills bind DNA but, in the absence of Hh, inhibits the pathway.
Phosphorylation events, which are mostly under the control of Cos2, mediate the control of the active and inactive form of Ci. Further, Sufu-Ci and Cos2-Fu-Ci complexes, in the absence of Hh, promote the formation of Ci-R and prevent its activation (Robbins et al., 1997; Sisson et al., 1997; Wang et al., 2000; Wang and Holmgren, 2000; Wang and Jiang, 2004; Zhang et al., 2004).
The Cos2-Fu-Ci complex communicates with the C-tail of Smo domains in the presence of Hh. Cos2 phosphorylation regulates this C-tail of Smo domains (Liu et al., 2007; Nybakken et al., 2002; Ranieri et al., 2012; Ranieri et al., 2014; Ruel et al., 2007) and promotes the formation of Ci-A and the resultant activation of the pathway.
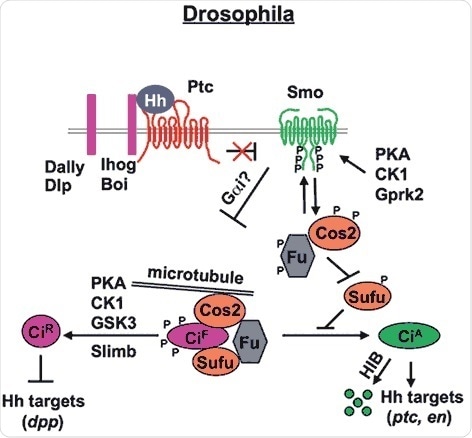
Figure 1. Drosophila Hh signal transduction pathway (Chen and Jiang, 2013). The mature Hh molecule reaches Hh receiving cells by binding with HSPGs, such as Dally and Dally-like (Dlp). In the absense of Hh, Ptc inhibits Smo allowing Ci to be phosphorylated by PKA, CK1 and GSK3. These phosphorylation events target Ci to a partial proteolytic cleavage (mediated by Slimb/β TRCP) to generate the repressor form (Ci-R). Binding of Hh to its receptor Ptc and co-receptor Ihog releases Ptc inhibition on Smo, which undergoes phosphorylation mainly by PKA and CK1. Consequently, Smo accumulates at the cell surface recruiting the Cos2-Fu-Ci complex. Here, according to the amount of Hh received by the cell, phosphorylation events on Cos2 and Fu regulate the activation of Ci and therefore of the pathway itself.
Hh signaling orthologues in vertebrates
Three paralogous Hh genes are present in mammals. They include:
- Indian hedgehog (Ihh, mainly involved in bone differentiation)
- Sonic hedgehog (Shh, the best studied and the most widely expressed Hh molecule)
- Desert hedgehog (Dhh, involved in gonad differentiation)
The key difference between Hh signaling in vertebrates and Drosophila is the need for the vertebrate intraflaggular transport (IFT) consisting of large multisubunits complexes that play a role in the bidirectional transport of proteins between the tip cilia and the base (Huangfu et al., 2003).
Both Smo and Ptc are capable of localizing to primary cilia in a mutually beneficial manner, where the binding between Ptc and Shh enables Smo to shift into the cilium and promotes the activation of the pathway via the Gli transcription factors (Rohatgi et al., 2007).
Following are the key differences and similarities between vertebrate and Drosophila Hh signaling:
- The Smo structure is highly preserved between vertebrates and Drosophila. The most interesting fact is that the phospho-sites on the Smo C-tail, as well as their dimerization mechanism, are also preserved, yet the kinases involved are somewhat different (Chen et al., 2011).
- Three Ci homologues called Gli1, Gli2 and Gli3. While Gli3 acts as a transcriptional repressor, the Gli1 and Gli2 functions as transcriptional activators (Ding et al., 1998; Matise et al., 1998; Park et al., 2000; Tempé et al., 2006).
- Vertebrate Sufu is different from Drosophila Sufu and plays an extremely critical role in the Shh pathway (Svärd et al., 2006), but both proteins share high sequence homology (Merchant et al., 2004; Stone et al., 1999).
- kif7 and kif27 – the Cos2 homologues – have preserved their negative role within the pathway by regulating the function and abundance of Gli (Cheung et al., 2009; Tay et al., 2005; Wilson et al., 2009).
- Mammalian Fu is capable of associating with kif27 and plays a role in ciliogenesis, whilst a compensatory Fu kinase, associated with kif7, is required for Hh signaling (Wilson et al., 2009).
The above factors indicate an evolutionary preservation in the Shh intracellular cascade, although more studies are required to gain a better understanding about the molecular mechanisms of the protein involved.
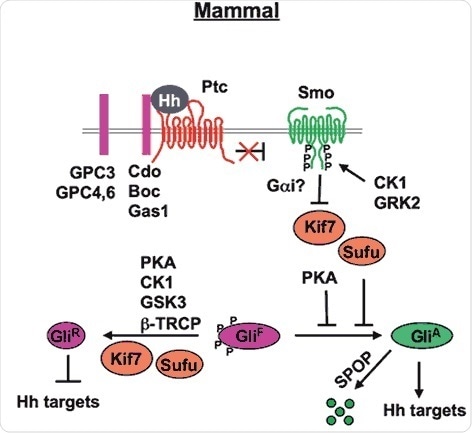
Figure 2. Mammal Hh signal transduction pathway (Chen and Jiang, 2013). The mature Hh molecule reaches Hh receiving cells by binding with HSPGs (such as GPC3, GPC4 and GPC6). In the absence of Hh, Ptc inhibits Smo allowing Gli to be phosphorylated by PKA, CK1 and GSK3. These phosphorylation events target Gli to a partial proteolytic cleavage (mediated by β-TRCP) to generate the repressor form (Gli-R). In the presence of Hh, binding of Hh to its receptor Ptc and co-receptor Cdo releases Ptc inhibition on Smo, which undergoes phosphorylation by mainly CK1 and GRK2. Consequently, Smo accumulates at the cell surface (within the cilia). Sufu is the major negative regulator of the pathway (kif7 is a minor one). In the presence of Hh, Sufu destabilization and degradation allows the release of its repression on Gli, with consequent pathway activation.
References
- Alexandre C, Jacinto A and Ingham PW (1996). Transcriptional activation of hedgehog target genes in Drosophila is mediated directly by the cubitus interruptus protein, a member of the GLI family of zinc finger DNA-binding proteins. Genes Dev 10, 2003–2013.
- Aza-Blanc P1, Ramírez-Weber FA, Laget MP, Schwartz C and Kornberg TB (1997). Proteolysis that is inhibited by hedgehog targets Cubitus interruptus protein to the nucleus and converts it to a repressor. Cell, 89, 1043–1053.
- Bolanos-Garcia VM and Miguel RN (2003). On the structure and function of apolipoproteins: more than a family of lipid-binding proteins. Prog Biophys Mol Biol, 83, 47–68.
- Burke R, Nellen D, Bellotto M, Hafen E, Senti KA, Dickson BJ and Basler K (1999). Dispatched, a novel sterol-sensing domain protein dedicated to the release of cholesterol-modified hedgehog from signaling cells. Cell, 99, 803–815.
- Chamoun Z, Mann RK, Nellen D, von Kessler DP, Bellotto M, Beachy PA and Basler K (2001). Skinny hedgehog, an acyltransferase required for palmitoylation and activity of the hedgehog signal. Science, 293, 2080–2084.
- Chen Y and Struhl G (1996). Dual roles for patched in sequestering and transducing Hedgehog. Cell, 1, 553–563.
- Chen Y, Sasai N, Ma G, Yue T, Jia J, Briscoe J and Jiang J (2011). Sonic Hedgehog dependent phosphorylation by CK1α and GRK2 is required for ciliary accumulation and activation of smoothened. PLoS Biol, 9, e1001083.
- Chen Y and Jiang J (2013). Decoding the phosphorylation code in Hedgehog signal transduction. Cell Res, 23, 186–200.
- Cheung HO, Zhang X, Ribeiro A, Mo R, Makino S, Puviindran V, Law KK, Briscoe J and Hui CC (2009). The kinesin protein Kif7 is a critical regulator of Gli transcription factors in mammalian hedgehog signaling. Sci Signal, 2, ra29.
- Claret S, Sanial M and Plessis A (2007). Evidence for a novel feedback loop in the Hedgehog pathway involving Smoothened and Fused. Curr Biol, 17, 1326–33.
- Denef N, Neubüser D, Perez L and Cohen SM (2000). Hedgehog induces opposite changes in turnover and subcellular localization of patched and smoothened. Cell, 18, 521–531.
- Ding Q, Motoyama J, Gasca S, Mo R, Sasaki H, Rossant J and Hui CC (1998). Diminished Sonic hedgehog signaling and lack of floor plate differentiation in Gli2 mutant mice. Development, 125, 2533–2543.
- Fan J, Liu Y and Jia J (2012). Hh-induced Smoothened conformational switch is mediated by differential phosphorylation at its C-terminal tail in a dose- and position-dependent manner. Dev Biol, 366, 172–184.
- Gallet A, Rodriguez R, Ruel L and Therond, PP (2003). Cholesterol modification of hedgehog is required for trafficking and movement, revealing an asymmetric cellular response to hedgehog. Dev Cell, 4, 191–204.
- Gallet A and Therond PP (2005). Temporal modulation of the Hedgehog morphogen gradient by a patched-dependent targeting to lysosomal compartment. Dev Biol, 277, 51–62.
- Hooper JE (2003). Smoothened translates Hedgehog levels into distinct responses. Development, 130, 3951–3963.
- Huangfu D, Liu A, Rakeman AS, Murcia NS, Niswander L and Anderson KV (2003). Hedgehog signalling in the mouse requires intraflagellar transport proteins. Nature, 426, 83–87.
- Ingham PW, Taylor AM and Nakano Y. (1991). Role of the Drosophila patched gene in positional signalling. Nature, 353, 184–187.
- Ingham PW and McMahon AP (2001). Hedgehog signalling in animal development: paradigms and principles. Genes Dev, 15, 3059–3087.
- Jia J, Tong C and Jiang J (2003). Smoothened transduces Hedgehog signal by physically interacting with Costal2/Fused complex through its C-terminal tail. Genes Dev, 17, 2709–2720.
- Khare N and Baumgartner S (2000). Dally-like protein, a new Drosophila glypican with expression overlapping with wingless. Mech Dev, 99, 199–202.
- Lee JD and Treisman JE (2001). Sightless has homology to transmembrane acyltransferases and is required to generate active Hedgehog protein. Curr Biol, 11, 1147–1152.
- Liu Y, Cao X, Jiang J and Jia J (2007). Fused-Costal2 protein complex regulates Hedgehog-induced Smo phosphorylation and cell-surface accumulation. Genes Dev, 21, 1949–1963.
- Lum L, Zhang C, Oh S, Mann RK, von Kessler DP, Taipale J, Weis-Garcia F, Gong R, Wang B and Beachy PA (2003). Hedgehog signal transduction via Smoothened association with a cytoplasmic complex scaffolded by the atypical kinesin, Costal-2. Mol Cell, 12, 1261–1274.
- Matise MP, Epstein DJ, Park HL, Platt KA and Joyner AL (1998). Gli2 is required for induction of floor plate and adjacent cells, but not most ventral neurons in the mouse central nervous system. Development, 125, 2759–2770.
- Merchant M, Vajdos FF, Ultsch M, Maun HR, Wendt U, Cannon J, Desmarais W, Lazarus RA, de Vos AM and de Sauvage FJ. (2004). Suppressor of fused regulates Gli activity through a dual binding mechanism. Mol Cell Biol, 24, 8627–8641.
- Méthot N and Basler K (1999). Hedgehog controls limb development by regulating the activities of distinct transcriptional activator and repressor forms of Cubitus interruptus. Cell, 96, 819–831.
- Nakato H, Futch TA and Selleck SB (1995). The division abnormally delayed (dally) gene: a putative integral membrane proteoglycan required for cell division patterning during postembryonic development of the nervous system in Drosophila. Development, 121, 3687–3702.
- Nusslein-Volhard C and Wieschaus E (1980). Mutation affecting segment number and polarity in Drosophila. Nature, 287, 795–801.
- Nybakken KE, Turck CW, Robbins DJ and Bishop JM (2002). Hedgehog-stimulated phosphorylation of the kinesin-related protein Costal2 is mediated by the serine/threonine kinase fused. J Biol Chem, 277, 24638–24647.
- Ogden SK, Ascano M Jr, Stegman MA, Suber LM, Hooper JE and Robbins DJ (2003). Identification of a functional interaction between the transmembrane protein Smoothened and the kinesin-related protein Costal2. Curr Biol, 13, 1998–2003.
- Olofsson SO, Asp L and Borén J (1999). The assembly and secretion of apolipoprotein B-containing lipoproteins. Curr Opin Lipidol, 10, 341–346.
- Park HL, Bai C, Platt KA, Matise MP, Beeghly A, Hui CC, Nakashima M and Joyner AL (2000). Mouse Gli1 mutants are viable but have defects in SHH signaling in combination with a Gli2 mutation. Development, 127, 1593–1605.
- Pepinsky RB, Zeng C, Wen D, Rayhorn P, Baker DP, Williams KP, Bixler SA, Ambrose CM, Garber EA, Miatkowski K, Taylor FR, Wang EA and Galdes A (1998). Identification of a palmitic acid modified form of human Sonic hedgehog. J Biol Chem, 273, 14037–14045.
- Porter JA, Young KE and Beachy PA (1996). Cholesterol modification of hedgehog signaling proteins in animal development. Science, 274, 255–259.
- Ranieri N, Ruel L, Gallet A, Raisin S and Thérond PP (2012). Distinct phosphorylations on kinesin costal-2 mediate differential hedgehog signaling strength. Dev Cell, 22, 279–294.
- Ranieri N, Thérond PP and Ruel L (2014). Switch of PKA substrates from Cubitus interruptus to Smoothened in the Hedgehog signalosome complex. Nat Commun, 5, 5034.
- Robbins DJ, Nybakken KE, Kobayashi R, Sisson JC, Bishop JM and Thérond PP (1997). Hedgehog elicits signal transduction by means of a large complex containing the kinesin-related protein costal2. Cell, 90, 225–234.
- Rohatgi R, Milenkovic L and Scott MP (2007). Patched1 regulates hedgehog signaling at the primary cilium. Science, 317, 372–376.
- Ruel L, Rodriguez R, Gallet A, Lavenant-Staccini L and Thérond PP (2003). Stability and association of Smoothened, Costal2 and Fused with Cubitus interruptus are regulated by Hedgehog. Nat Cell Biol, 5, 907–913.
- Ruel L, Gallet A, Raisin S, Truchi A, Staccini-Lavenant L, Cervantes A and Thérond PP (2007). Phosphorylation of the atypical kinesin Costal2 by the kinase Fused induces the partial disassembly of the Smoothened-Fused-Costal2-Cubitus interruptus complex in Hedgehog signalling. Development, 134, 3677–3689.
- Sisson JC, Ho KS, Suyama K and Scott MP (1997). Costal2, a novel kinesin-related protein in the Hedgehog signaling pathway. Cell, 90, 235–245.
- Slusarski DC, Motzny CK and Holmgren R (1995). Mutations that alter the timing and pattern of cubitus interruptus gene expression in Drosophila melanogaster. Genetics, 139, 229–240.
- Stegman MA, Vallance JE, Elangovan G, Sosinski J, Cheng Y and Robbins DJ (2000). Identification of a tetrameric hedgehog signaling complex. J Biol Chem, 275, 21809–21812.
- Stone DM, Murone M, Luoh S, Ye W, Armanini MP, Gurney A, Phillips H, Brush J, Goddard A, de Sauvage FJ and Rosenthal A (1999). Characterization of the human suppressor of fused, a negative regulator of the zinc-finger transcription factor Gli. J Cell Sci, 112, 4437–4448.
- Svärd J, Heby-Henricson K, Persson-Lek M, Rozell B, Lauth M, Bergström A, Ericson J, Toftgård R and Teglund S (2006). Genetic elimination of Suppressor of fused reveals an essential repressor function in the mammalian Hedgehog signaling pathway. Dev Cell, 10, 187–197.
- Tay SY, Ingham PW and Roy S (2005). A homologue of the Drosophila kinesin-like protein Costal2 regulates Hedgehog signal transduction in the vertebrate embryo. Development, 132, 625–634.
- Tempé D, Casas M, Karaz S, Blanchet-Tournier MF and Concordet JP (2006). Multisite protein kinase A and glycogen synthase kinase 3beta phosphorylation leads to Gli3 ubiquitination by SCFbetaTrCP. Mol Cell Biol, 26, 4316–4326.
- Wang G, Amanai K, Wang B and Jiang J (2000). Interactions with Costal2 and suppressor of fused regulate nuclear translocation and activity of cubitus interruptus. Genes Dev, 14, 2893–2905.
- Wang G and Jiang J (2004). Multiple Cos2/Ci interactions regulate Ci subcellular localization through microtubule dependent and independent mechanisms. Dev Biol, 268, 493–505.
- Wang QT and Holmgren RA (2000). Nuclear import of cubitus interruptus is regulated by hedgehog via a mechanism distinct from Ci stabilization and Ci activation. Development, 127, 3131–3139.
- Wilson CW, Nguyen CT, Chen MH, Yang JH, Gacayan R, Huang J, Chen JN and Chuang PT (2009). Fused has evolved divergent roles in vertebrate Hedgehog signalling and motile ciliogenesis. Nature, 459, 98–102.
- Zhang C, Williams EH, Guo Y, Lum L and Beachy PA (2004). Extensive phosphorylation of Smoothened in Hedgehog pathway activation. Proc Natl Acad Sci USA, 101, 17900–17907.
- Zhang W, Zhao Y, Tong C, Wang G, Wang B, Jia J and Jiang J (2005). Hedgehog-regulated Costal2-kinase complexes control phosphorylation and proteolytic processing of Cubitus interruptus. Dev Cell, 8, 267–278.
- Zhao Y, Tong C and Jiang J (2007). Hedgehog regulates smoothened activity by inducing a conformational switch. Nature, 450, 252–258.
- Zheng X, Mann RK, Sever N and Beachy PA. (2010). Genetic and biochemical definition of the Hedgehog receptor. Genes Dev, 24, 57–71.
About Abcam
Abcam is a global life sciences company providing highly validated antibodies and other binders and assays to the research and clinical communities to help advance the understanding of biology and causes of disease.
Abcam’s mission is to serve life scientists to help them achieve their mission faster by listening to their needs, continuously innovating and improving and by giving them the tools, data and experience they want. Abcam’s ambition is to become the most influential life science company for researchers worldwide.
Sponsored Content Policy: News-Medical.net publishes articles and related content that may be derived from sources where we have existing commercial relationships, provided such content adds value to the core editorial ethos of News-Medical.Net which is to educate and inform site visitors interested in medical research, science, medical devices and treatments.