Retinol is a type of vitamin A. Both retinol and its metabolites are central to a wide range of physiological processes. These include vision, immune response regulation, the differentiation of cells, and development in vertebrates. The bioactive metabolites of vitamin A that have been best characterized are 11-cis-retinal and all-trans-retinoic acid (ATRA, 1). Photoreception is mediated by the 11-cis-retinal metabolite by working as the visual chromophore.1 The majority of the non-visual functions of vitamin A are mediated by ATRA,2 which regulates the expression of specific subsets of genes within target tissues via nuclear receptors.3
Retinoic Acid and Retinoid X Receptors
Nuclear receptors are ligand-activated transcription factors that bind to certain DNA regulatory elements in the promoter or vicinity of target genes that lead to transcriptional activation or repression. The structures of nuclear receptors are composed of several domains labeled A to F sequentially from the amino terminus and can be described as modular.
The amino terminal A/B comprises the ligand-independent transcriptional activation function, AF-1, which imparts promoter specificity, while the C domain contains the DNA binding domain (DBD). The E/F carboxy terminal domain contains the ligand-binding domain (LBD) and is separated from the C domain by a flexible hinge region (D).
ATRA binds the LBD of one of three isotypes of the retinoic acid receptor (RAR), namely RARα, β, or γ.3–6 RAR creates heterodimers with one of three isotypes of the retinoid X receptor (RXR): RXRα, β or γ. 4,5 Although ATRA doesn’t bind or activate RXRs, its 9-cis isomer can bind and activate (2).7,8 The RAR-RXR heterodimer associates with retinoic acid response elements (RARE).
These elements are made up of a direct repeat (DR) of a stretch of DNA that corresponds to the canonical sequence 5′-PuG(G/T)TCA. Often, five nucleotides (DR5) separate the half-sites of a RARE, but sometimes only two (DR2) can separate the half-sites.9 On the direction of the target gene’s transcription about the RAR-RXR arrangement, RXR is found upstream of its RAR partner (5′-RXR-RAR-3′).
Binding an agonist to RAR overcomes the role of agonist binding to RXR. As a result, RARRXR is classed as a non-permissive heterodimer. In addition to RAR, RXR is also a heterodimeric partner of a number of nuclear receptors, which include both nonpermissive partners, like the thyroid receptor, vitamin D3 receptor and pregnane X receptor, and permissive partners like peroxisome proliferator-activated receptors (PPARs) α, β/δ, and γ, the farnesoid X receptor (FXR) and liver X receptor (LXR).
Within the framework of permissive heterodimeric partners, agonist binding may well influence the functions of these heterodimeric complexes. When bound to an agonist, the RAR heterodimeric partner goes through a conformational change in the helical motif H12 (referred to as AF-2). This enables RAR to couple to co-activator proteins responsible for mediating transcriptional effects.10,11
The best characterized nuclear receptor co-activators are part of the steroid receptor co-activator (SRC-1) family. SRC-1 is needed for ligand-dependent transcription of transiently transfected and chromosomally integrated reporter genes by the estrogen receptor (ER) and RAR.12,13 Co-activators interact with nuclear receptors bound to ligands via a central domain that holds three copies of a conserved recognition motif with the consensus sequence LXXLL.14
Ligands are not always necessary for the RAR-RXR heterodimer to bind RARE. However, it is more likely to work with co-repressors like the nuclear receptor co-repressor (N-CoR) 15 proteins and silencer proteins, silencing mediator for retinoid and thyroid receptor (SMRT), for example.16
These corepressors take on histone deacetylase and methyl transferase complexes resulting in chromatin condensation and sequestration of promoter elements, which impede transcriptional activity.
Along with the traditional ATRA-RAR signaling pathway, there are alternative, naturally occurring retinol metabolites have proven to demonstrate biological activities in vitro, like all-trans-13,14-dihydroxy-retinol and the retro-retinoids, specifically anhydroretinol (AR) and 14-hydroxy-4,14- retro-retinol (14-HRR), both of which are involved in lymphocyte proliferation regulation.17–20
Meanwhile, saturation of all-trans-retinol by the all-trans-13,14-dihydroretinol saturase (RetSat) enzyme results in the formation of (13R)-all-trans-13,14- dihydroretinol21 that can be further oxidized to (13R)-all-trans-13,14-dihydroretinoic acid22. This is a compound displaying RAR agonist activity.23
This range of metabolites and their activities suggest that there are other ATRA-independent functions of vitamin A that are yet to be discovered. For every RAR or RXR isotype, there are numerous variants produced by alternative splicing or alternate transcriptional start sites. Each of the three RXR isotypes carry two isoforms, which can also be said for RARα and γ. RARβ, however, carries four isoforms.
48 distinct heterodimers can be produced as a result of the combinations of 8 RAR and 6 RXR isoforms and isotypes, and a single endogenous ligand’s capacity to modulate an array of specific genes both temporally and spatially can be elucidated by the numerous possible heterodimers. Genetic ablation of gene coding expression for a single isotype (like mice lacking expression of RARα (Rara-null) for example) can be compensated for in vivo by the remaining isotypes to some extent. This is true of RARβ (Rarb), or RARγ (Rarg) in this particular case.24
A further level of complexity has been added to our understanding of the roles of these remaining isotypes by their redundant and overlapping functions. These isotypes have also complicated our understanding of the development of isotype-selective drugs.
Pharmacology of RAR and RXR
Pharmacologically, both RAR and RXR can be modulated in a subtype-specific manner with agonists, inverse agonists, partial agonists, and antagonists. Both naturally occurring and synthetic modulators of RARs are known as retinoids.
Naturally occurring and synthetic modulators of RXR are known as rexinoids. Retinoids do possess significant toxicity, which has to be taken into account when retinoids are considered for use in therapy. Such toxicity includes teratogenesis, the induction of the retinoic acid syndrome characterized by weight gain, elevated white blood cells (WBCs), respiratory distress, interstitial pulmonary infiltrates, pleural and pericardial effusion, shortness of breath, episodes of low blood pressure, and acute renal failure (ARF).
By creating more receptor-selective agents, the harmful effects could be successfully avoided while also gaining specific responses and therapeutic worth. Retinoid signaling can trigger cellular differentiation or cell death, which is particularly pertinent in cancer treatments. As a result, there has been a concerted effort to create safe and receptor-selective compounds.
Unprecedented molecular understanding of the nuanced changes necessary to create the receptor response and to confer specific activity has been achieved through structural analysis of a range of receptors in combination with ATRA, 9-cis-RA or synthetic agonists or antagonists. Unfortunately, at the time of writing, a ligand-free structure of RAR is not available to enable us to comprehend the mechanism by which activation occurs fully.
Several expert reviews have thoroughly discussed the design strategy, approach and history of retinoid research.25–29
Several diseases and disorders have seen retinoids used in their treatment. Supplementation with all-trans-retinol is used in developing countries to improve vitamin A deficiency (VAD), which is an example of one of the safest and most effective therapeutic uses of retinoids that have enormous global impact, saving millions of lives every year.30 Additionally, all-trans-RA (tretinoin) and 13-cis-RA (isotretinoin, 3) are used to treat severe cases of acne among other dermatological conditions.31
In cancer settings, the activation of RAR signaling results in cell differentiation and has chemopreventative and chemotherapeutic effects. In Acute Promyelocytic Leukemia (APL), a fusion of RAR with PML (promyelocytic leukemia protein) growth suppressor gene affects RAR signaling. Retinoid agonists, for example ATRA, can reverse the effects of PML-RAR.
As well as triggering cell differentiation, certain retinoids cause tumor cell death and provide another chemotherapeutic mechanism. Retinoids including ATRA, 13-cis-RA, N-(4-hydroxyphenyl)retinamide (4-HPR or fenretinide, 4) and synthetic subtype-selective RAR and RXR agonists and modulators are employed in the treatment and chemoprevention of various cancers.32–35
The majority of retinoids and rexinoids are similar to the retinoic acid scaffold and employ a carboxylic acid moiety to make a critical ionic bridge with an arginine residue within the ligand-binding domain of the respective receptors.
RAR Agonists and Antagonists
Pan-RAR modulators approved in the clinic include ATRA (tretinoin) and its 9-cis (alitretinoin) and 13-cis (isotretinoin) isomers. Both ATRA and 13-cis compounds operate as pan-RAR agonists, while 9-cis-RA can operate as a pan-RAR and pan-RXR agonist. Both 9-cis and 13-cis-RA can isomerize to ATRA (the more stable isomer) in vivo and can be degraded by the enzymes that also regulate ATRA levels.
EC 23 (5), a stable analog of ATRA which doesn’t isomerize, has demonstrated powerful activity in the induction of stem cells and neurogenesis.36,37 Other potent pan-RAR agonists include the arotinoid TTNPB (6), which was crystallized in association with RARβ, 38 and the synthetic chalconoid Ch 55 (7).39 Tazarotene (8), another stable RAR analog, shows specificity towards RARβ and RARγ40 and is currently used as a topical acne and psoriasis treatment.41
RARα-specific agonists include BMS 753 (9),42 AM 80 (10) and its related compound AM 580 (11).43 The structure of RARα-LBD in association with a co-activator fragment and complexed with AM 580 has also been recently determined.44 These studies demonstrate that AM 580 reduces the affinity of all co-repressor motifs for RAR, and describe the structural changes necessary for the repositioning of H12, allowing the release of co-repressors and the recruitment of co-activators.
RARβ expression is regularly lost in many transformed cells, meaning RARβ agonists would need to have potent antiproliferative effects. RARβ-specific agonists include the 4′-octyl-4-biphenylcarboxylic acid, AC 55649 (12), discovered through functional high throughput screening, and AC 261066 (13), developed by optimizing AC 55649.45,46 Adapalene (14), which carries the 1-adamantyl substituent, displays agonistic activity against both RARβ and RARγ, and is used in acne treatment. 47,48
RARγ-specific agonists include adapalene derivatives CD 437 (15) and CD 1530 (16).49 The pro-apoptotic and antitumor effects of CD 437 are believed to work independently of the interaction with RARγ50,51, and possibly through proapoptotic mitochondrial pathway modulation52,53 or GADD45A protein.54 A different adapalene derivative, CD 2665 (17), displays antagonistic activities against both RARβ and RARγ. 49,52
In general, inserting a bulky substituent can change an agonist into an antagonist, as shown by the pan-RAR antagonist MM 11253 (18) 55 and the RARβ antagonist LE 135 (19).56 The stilbene arotinoids BMS 493 (20) and BMS 453 (21) have an intriguing profile characterized by either inverse agonist or mixed agonist/antagonist activities. BMS 453 is a strong antagonist of TTNPB-induced activation of RARα or RARγ, and a pan-RAR inverse agonist that boosts the RAR interaction with co-repressors down-regulating RAR-mediated gene transcription.44 The newly discovered pan-RAR inverse agonist SR-0065 embodies the first non-acid, non-retinoid direct modulator of the RAR receptor subfamily.58
RXR Agonists and Antagonists
Development of isotype selective rexinoids has proved more of a challenge due to the highly conserved nature of the residues that delineate the lipid-binding pocket of RXR.25,59 From a subtype perspective, RXRα is the main RXR isotype involved in the formation and development of embryos,6 and RXRα-selective agonists are relatively uncommon. Furthermore, activation of RXR alone is not expected to result in transcription activation and precludes the use of rexinoid monotherapy to modulate RAR signaling because of the non-permissive nature of the RAR-RXR heterodimer.
Activating RXR in the presence of RAR agonists can lead to synergistic effects and translate into stronger activity. Conversely, RXR activation in the context of permissive heterodimers generally leads to transcription activation. As a result, the use of RXR agonists may lead to pleiotropic effects by activating multiple permissive heterodimeric complexes. The huge array of RXR permissive partners provides a potentially significant pharmacological tool for use in targeting multiple receptors and pathways that converge to gain a constructive pharmaceutical profile.
Dual activation of PPARα and PPARγ results in heightened fatty acid oxidation and fat recruitment into adipose stores; both pathways contribute to reducing the levels of ectopic fat and circulating fatty acids and lead to higher insulin sensitivity.60 Such effects were seen very early using the panRXR agonist bexarotene (22).61 However, potent pan-RXR agonists can also bring on RXR homodimer-dependent transcription, which can result in detrimental effects triggered by thyroid axis suppression.62 Contrary to this are heterodimer-selective rexinoids such as LG 101506 (23), which bring on insulin sensitization and do not suppress thyroid signaling.63
Other pan-RXR agonists include the pan-RAR/pan-RXR agonist, 9-cis-retinoic acid, and benzoic acid derivatives CD 3254 (24) 64 and SR 11237 (BMS 649, 25), which was co-crystallized with RXRα and a co-activator-derived peptide.59 The related compound UVI 3003 (26) operates as a pan-RXR antagonist and is a valuable tool for dissecting the contribution of RXR to transactivation in heterodimers, particularly in permissive heterodimers.64
Another benzoic acid derived RXR ligand, LG 100754, has also been crystallized in association with RXRα and categorized as an RXR homodimer antagonist and a selective pan-RXR heterodimer agonist.
LG 100754 (27) works as an agonist of PPARα/RXR, but it doesn’t activate the thyroid receptor (TR)/RXR, vitamin D receptor (VDR)/RXR or LXR/RXR heterodimers.65,66 The paradoxical result of an RXR antagonist like LG 100754 operating as an activator of RAR-RXR heterodimers was clarified though a ‘phantom ligand’ effect, whereby binding LG 100754 to RXR translates into conformational change in RAR and recruitment of co-activators.67
However, more recent studies show that antagonism of RXR by LG 100754 does not affect the structure of RAR or its interaction with co-repressors or co-activators within the heterodimer. Instead, LG 100754 mediates RAR activation through direct binding.68 Consequently, LG 100754 still operates as an RXR antagonist in the context of RARRXR heterodimers.68
Similar to LG 101506, LG 100754 is also a potent insulin-sensitizer.66 In contrast, the dibenzodiazepine derivative HX 531 (28) is a pan-RXR antagonist that impedes both RXR homodimers and RAR-RXR heterodimer activation. Specifically, HX 531 impeded the transactivation of RARs brought on by the RAR agonist, AM 80.69
An associated derivative of dibenzodiazepine, HX 630 (29), works as a pan-RXR agonist and weak RAR-antagonist.70 Both HX 630 and HX 531 display weak binding affinity to isolated RXR (with submicromolar Ki values) while showing stronger agonist or antagonist activity, respectively, at RAR-RXR heterodimers (with subnanomolar EC50 values) suggesting that they are RXR-RAR heterodimer-selective ligands.70
The structure of RXR, in combination with an array of agonists showed that the RXR lipid-binding pocket is more cooperative than the one of RAR, enabling RXR to bind several ligands like the proposed endogenous ligand, 9-cis-RA, as well as other long-chain, unsaturated fatty acids.
It could be argued that RXR doesn’t have a fully dedicated endogenous ligand. It could also be said that it evolved to respond to several molecular signatures depending on the cellular and metabolic context and the local concentration and supply of each potential ligand. Utilizing genetic and pharmacological evidence, it has been possible to eliminate a physiological role for 9-cis-RA as an RXR ligand in mouse keratinocytes.71
Taken together with RXR’s opportunistic nature, this disputes the nature of its endogenous ligand. Before it was recently detected in the pancreas of mice by employing the use of a sensitive analytical LC-MS/MS technique,72 9-cis-RA couldn’t be distinguished in animal tissue unless they were supplemented with pharmacological doses of vitamin A beforehand.
The difficulties experienced in detecting endogenous 9-cis-RA inspired the search for alternate ligands. Other potential endogenous RXR ligands include docosahexaenoic acid (DHA),73 the most widely found essential omega-3 fatty acid in the brain, and phytanic acid, created from the degradation of chlorophyll by α-oxidation.74 Both DHA and phytanic acid have an array of alternative potential cellular receptors or mediators that preclude their roles as selective rexinoids.
DHA can also activate PPARγ75 and can display several other effects, (reviewed by Niemoller et al.76) while phytanic acid can also activate PPARα.77
Off-target Effects of Retinoids and Rexinoids
One significant consideration in retinoid use and rexinoids is the possibility for off-target effects. Our understanding of retinoid and rexinoid behavior can sometimes be limited to in vitro assays where the response of an isolated receptor is investigated one ligand at a time. The use of such compounds in cell-based assays or in vivo (entirely based on reported in vitro activity) is rife with the potential for off-target effects as evidenced in the widely used RARα-specific antagonist Ro 41-5253, which is also an agonist of PPARγ.78
Current methodologies enable researchers to screen a ligand for agonistic or antagonistic effects against an extensive panel of nuclear receptors, thereby lowering the risk of off-target effects. In one study, screening 25 human nuclear receptors enabled the researchers to discover that all-trans-13,14-dihydroretinoic acid displays RAR-specificity.23
When creating ranges of improved drugs, a solid understanding of retinoid and rexinoid pharmacology beyond RAR and RXR – and screening for off-target effects is vital. Off-target effects may provide alternative research areas for retinoid compounds with known antitumor activity, for example, atypical retinoids, which have been evidenced to bind and activate RAR or RXR in vitro.
However, this does not entirely explain their antitumor in vivo effects. Considering CD 437 and fenretinide, both seem to be effective in retinoid-resistant cells and their effects cannot be blocked with RAR antagonists.79 Fenretinide does not possess the carboxylic acid that would enable it to cooperate with the RAR LBD but can be converted to ATRA. Although, this is not a requirement for triggering cell death.80 Both drugs are promising therapeutic agents.
CD 437 and fenretinide are currently in preclinical studies for their use in various cancer treatments. Additional to activating the RAR/RXR heterodimer, ATRA has also been proposed to modulate cell signaling through RAR independent pathways (nonclassical pathways) that have been found to occur in many settings. Such settings include neural development,81 mucous cell differentiation82, and the maturation of dendritic cells.83
Several receptor molecules have been proposed to mediate the non-classical effects of ATRA, from non-RAR nuclear transcription factors like the retinoic acid orphan receptor γ (RORγ),84 to PPARβ/δ,85 testicular receptor 4 (TR4)86, and chicken ovalbumin upstream promoter-transcription factors II (COUPTFII)87. Additional to these, other cellular signaling mediators have also been proposed to mediate non-classical ATRA effects, like the mannose-6-phosphate receptor/IGF2R88,89 and extracellular signal-regulated kinase (ERK).
Even though RAR is thought of as a classical nuclear receptor, it still reveals new sides of its complexity. Its full potential may not have yet been fully determined. RARα, in response to ATRA, can carry out transcription-independent effects through the regulation of the translation of neuronal proteins like the glutamate receptor 1 (GluR1) mRNA, and mediating synaptic plasticity.90–93
Modulators of ATRA Metabolism
Retinoid signaling is controlled through the isotype of nuclear receptor present, the levels of nuclear receptors, and the levels of ligand available. Both excess and deficiency of ligand can result in severe developmental abnormalities. The levels of ATRA are intricately well-controlled in vivo both temporally and spatially by the enzymes and binding proteins taking part in its synthesis and catabolism.
The enzymes playing a part in retinoic acid’s synthesis and degradation have been widely documented. Retinol (ROL) and retinaldehyde (RAL) can be interconverted by microsomal short-chain dehydrogenase/reductase (SDR)94,95 and by class I, II, and IV medium-chain alcohol dehydrogenases (ADH).96 Irreversible oxidation of RAL to RA is carried out by retinal dehydrogenase (RALDH) types 1, 2, 3, and 4.97–102 Cytochrome P450 enzymes CYP26A1, CYP26B1, and CYP26C1 carry out the catabolism of RA to 4-hydroxy-RA, 4-oxoRA, and 18-hydroxy-RA.103–106
Retinoids are easily altered and are not particularly soluble in aqueous solutions. Transport proteins are a key element in vivo in that they shield retinoids from oxidation or isomerization and make sure they are delivered to target tissues, enzymes or receptors.107 Transport of retinol in serum is mediated by retinol-binding protein (RBP)108 which circulates bound to transthyretin (TTR).109
Cellular uptake and efflux of retinol takes place through receptors like receptors stimulated by retinoic acid 6 (STRA6), located on vascular-endothelial cells and retinal pigmented epithelial cells.110–112 Cellular uptake progresses by retinol esterification by lecithin:retinol acyltransferase enzymes to create extremely insoluble retinyl esters.
ATRA is delivered to the nucleus by accessory proteins CRABP (cellular retinoic acid-binding proteins) I and II, soluble proteins from the intracellular ligand-binding protein family (iLBPs).113 Both CRABPI and II remain in the cytosol without the presence of a ligand. However, CRABPI and II translocate to the nucleus in the presence of a ligand. CRABPII delivers the ligand by interacting directly with RARs114 and stimulating their activity.115
The type of accessory protein can control the ligand’s fate. For example, retinoic acid, which can be transported to the nucleus by CRABPI or II or fatty acid-binding protein 4 (FABP4 or aP2) to bind either RAR or PPARβ/δ respectively, according to which accessory protein is present.116 Therefore, binding a specific ligand to alternate receptors can be modulated by the presence of specific accessory molecules.
This is an important point to consider when investigating a ligand’s activity, either in vivo or in cell culture. Modulation of retinoid metabolism or transport can affect the precursor pool of ATRA or result in pharmacokinetic enhancement of ATRA. Inhibitors of the CYP26A1 enzymes such as liarozole hydrochloride trigger a rise in ATRA levels in cells, leading to augmentation of RAR signaling in pan-RAR fashion.
Fenretinide, in addition to its effects encouraging apoptosis, interrupts RBP binding to transthyretin, which leads to RPB excretion via glomerular filtration and causes a decrease in the precursor pool of retinol for the synthesis of ATRA.117
Conclusion
Advances in structural biology began with the clarification of an RXR fragment that encompassed the LBD,118 then the ligand-bound LBD of RARs119 and the DBD of RAR-RXR on DNA.120 After this, several structures showing the RAR or RXR LBD complexed with agonists, antagonists and co-activator and corepressor peptides (reviewed by de Lera et al.25) followed. This has resulted in a deeper understanding of the molecular details of receptor activation, repression, and the role of specific domains in the association with co-activator and co-repressor.
Due to the unstructured and flexibility of the hinge separating the DBD and LBD, there is a lack of full-length receptor structures. The clarification of the structure of an intact receptor, containing both the LBD and DBD, has become a vital move in understanding how the two domains interact and how activation translates into global alterations.
The first crystal structure of a full-length nuclear receptor complex on DNA was reported by Chandra et al.121 demonstrating the assembly of full-length PPARγ-RXRα heterodimers on DNA. This level of understanding regarding RAR-RXR will allow improvements in the selectivity of retinoids and rexinoids, as well as the potential for the development of allosteric modulators.
These allosteric modulators would probably not be based on the retinoid scaffold and would bind to a separate site from the LBD to mediate the interaction of nuclear receptors with co-activators and co-repressors in a ligand-dependent manner. Allosteric modulators would also need to work in a more isotype-selective manner when compared to current retinoids and rexinoids, which are limited to bind the highly conserved LBD.
Unfortunately, the development of allosteric modulators generally takes place through structure-guided design or fragment-based drug discovery efforts. Both approaches have been found to place significant challenges in the way of nuclear receptors as they need high-resolution structures of intact receptors and highly reproducible crystallization conditions. In the future, the incorporation of screens for co-activator binding and structural biology could enable new classes of modulators and substantial progress in the retinoid and rexinoid field.
Special Mentions
This white paper was based on the original work of:
Alexander R. Moise: Alexander Moise is an Assistant Professor at the University of Kansas, School of Pharmacy and a Pilot Project leader of the NIH Center of Biomedical Research Excellence in Protein Structure and Function (COBRE-PSF) at the University of Kansas. His research focuses on the role of lipid mediators in health and disease and he is currently characterizing lipid-processing enzymes as therapeutic targets in visual diseases, obesity and cancer.
Department of Pharmacology & Toxicology, School of Pharmacy,University of Kansas, USA.
Email: [email protected]
References and Further Reading
- Wald (1968) Molecular basis of visual excitation. Science 162 230.
- Dowling and Wald (1981) Nutrition classics. Proceeding of the National Academy of Sciences of the United States of America, Volume 46, 1960: The biological function of vitamin A acid. Nutr.Rev. 39 13.
- Chambon (1996) A decade of molecular biology of retinoic acid receptors. FASEB J. 10 940.
- Petkovich et al. (1987) A human retinoic acid receptor which belongs to the family of nuclear receptors. Nature 330 444.
- Giguere et al. (1987) Identification of a receptor for the morphogen retinoic acid. Nature 330 624.
- Mark et al. (2006) Function of retinoid nuclear receptors: Lessons from genetic and pharmacological dissections of the retinoic acid signalling pathway during mouse embryogenesis. Annu.Rev.Pharmacol.Toxicol. 46 451.
- Heyman et al. (1992) 9-cis retinoic acid is a high affinity ligand for the retinoid X receptor. Cell 68 397.
- Egea et al. (2000) Crystal structure of the human RXRα ligand-binding domain bound to its natural ligand: 9-cis retinoic acid. EMBO J. 19 2592.
- Predki et al. (1994) Ordered binding of retinoic acid and retinoid-X receptors to asymmetric response elements involves determinants adjacent to the DNA-binding domain. Mol.Endocrinol. 8 31.
- Darimont et al. (1998) Structure and specificity of nuclear receptorcoactivator interactions. Genes.Dev. 12 3343.
- Nolte et al. (1998) Ligand binding and co-activator assembly of the peroxisome proliferator-activated receptor-γ. Nature 395 137.
- Onate et al. (1995) Sequence and characterization of a coactivator for the steroid hormone receptor superfamily. Science 270 1354.
- Llopis et al. (2000) Ligand-dependent interactions of coactivators steroid receptor coactivator-1 and peroxisome proliferator-activated receptor binding protein with nuclear hormone receptors can be imaged in live cells and are required for transcription. Proc.Natl.Acad.Sci.USA 97 4363.
- Ding et al. (1998) Nuclear receptor-binding sites of coactivators glucocorticoid receptor interacting protein 1 (GRIP1) and steroid receptor coactivator 1 (SRC-1): multiple motifs with different binding specificities. Mol.Endocrinol. 12 302.
- Kurokawa et al. (1995) Polarity-specific activities of retinoic acid receptors determined by a co-repressor. Nature 377 451.
- Chen and Evans (1995) A transcriptional co-repressor that interacts with nuclear hormone receptors. Nature 377 454.
- Buck et al. (1991) Intracellular signaling by 14-hydroxy-4,14-retroretinol. Science 254 1654.
- Buck et al. (1993) Anhydroretinol: a naturally occurring inhibitor of lymphocyte physiology. J.Exp.Med. 178 675.
- Derguini et al. (1994) Intracellular signaling activity of synthetic (14R)-, (14S)-, and (14RS)-14-hydroxy-4,14-retro-retinol. Biochemistry 33 623.
- Derguini et al. (1995) 13,14-Dihydroxy-retinol, a new bioactive retinol metabolite. J.Biol.Chem. 270 18875.
- Moise et al. (2004) Identification of all-trans-retinol:all-trans-13,14-dihydroretinol saturase. J.Biol.Chem. 279 50230.
- Moise et al. (2005) Metabolism and transactivation activity of 13,14-dihydroretinoic acid. J.Biol.Chem. 280 27815.
- Moise et al. (2009) Activation of retinoic acid receptors by dihydroretinoids. Mol.Pharmacol. 76 1228.
- Lufkin et al. (1993) High postnatal lethality and testis degeneration in retinoic acid receptor α mutant mice. Proc.Natl.Acad.Sci.USA 90 7225.
- de Lera et al. (2007) Design of selective nuclear receptor modulators: RAR and RXR as a case study. Nat.Rev.Drug Discov. 6 811.
- Altucci et al. (2007) RAR and RXR modulation in cancer and metabolic disease. Nat.Rev.Drug Discov. 6 793.
- Germain et al. (2006) International Union of Pharmacology. LX. Retinoic acid receptors. Pharmacol.Rev. 58 712.
- Dawson (2004) Synthetic retinoids and their nuclear receptors. Curr.Med.Chem.Anticancer Agents 4 199.
- Thacher et al. (2000) Therapeutic applications for ligands of retinoid receptors. Curr.Pharm.Des. 6 25.
- Humphrey et al. (1992) Vitamin A deficiency and attributable mortality among under-5-year-olds. Bull.World Health Organ. 70 225.
- Chivot (2005) Retinoid therapy for acne. A comparative review. Am.J.Clin.Dermatol. 6 13.
- Chomienne et al. (1989) Retinoic acid therapy for promyelocytic leukaemia. Lancet 2 746.
- Hong and Sporn (1997) Recent advances in chemoprevention of cancer. Science 278 1073.
- Simeone and Tari (2004) How retinoids regulate breast cancer cell proliferation and apoptosis. Cell.Mol.Life Sci. 61 1475.
- Hail et al. (2006) Mechanisms of fenretinide-induced apoptosis. Apoptosis 11 1677.
- Christie et al. (2010) Retinoid supplementation of differentiating human neural progenitors and embryonic stem cells leads to enhanced neurogenesis in vitro. J.Neurosci.Methods 193 239.
- Christie et al. (2008) Synthesis and evaluation of synthetic retinoid derivatives as inducers of stem cell differentiation. Org.Biomol.Chem. 6 3497.
- Germain et al. (2004) Rational design of RAR-selective ligands revealed by RARβ crystal structure. EMBO Rep. 5 877.
- Nervi et al. (1989) Identification and characterization of nuclear retinoic acid-binding activity in human myeloblastic leukemia HL-60 cells. Proc.Natl. Acad.Sci.USA 86 5854.
- Chandraratna (1996) Tazarotene - first of a new generation of receptorselective retinoids. Br.J.Dermatol. 135 (Suppl 49) 18.
- Talpur et al. (2009) Efficacy and safety of topical tazarotene: a review. Expert.Opin.Drug Metab.Toxicol. 5 195.
- Gehin et al. (1999) Structural basis for engineering of retinoic acid receptor isotype-selective agonists and antagonists. Chem.Biol. 6 519.
- Kagechika et al. (1988) Retinobenzoic acids. 1. Structure-activity relationships of aromatic amides with retinoidal activity. J.Med.Chem. 31 2182.
- le Maire et al. (2010) A unique secondary-structure switch controls constitutive gene repression by retinoic acid receptor. Nat.Struct.Mol.Biol. 17 801.
- Lund et al. (2005) Discovery of a potent, orally available, and isoformselective retinoic acid β2 receptor agonist. J.Med.Chem. 48 7517.
- Lund et al. (2009) Design, synthesis, and structure-activity analysis of isoform-selective retinoic acid receptor β ligands. J.Med.Chem. 52 1540.
- Hensby et al. (1990) The in vivo and in vitro anti-inflammatory activity of CD271: a new retinoid-like modulator of cell differentiation. Agents Actions 29 56.
- Bernard (1993) Adapalene, a new chemical entity with retinoid activity. Skin Pharmacol. 6 61.
- Bernard et al. (1992) Identification of synthetic retinoids with selectivity for human nuclear retinoic acid receptor γ. Biochem.Biophys.Res.Commun. 186 977.
- Shao et al. (1995) p53 independent G0/G1 arrest and apoptosis induced by a novel retinoid in human breast cancer cells. Oncogene 11 493.
- Sun et al. (2000) Dual mechanisms of action of the retinoid CD437: nuclear retinoic acid receptor-mediated suppression of squamous differentiation and receptor-independent induction of apoptosis in UMSCC22B human head and neck squamous cell carcinoma cells. Mol.Pharmacol. 58 508.
- Sun et al. (1997) Induction of apoptosis in human non-small cell lung carcinoma cells by the novel synthetic retinoid CD437. J.Cell.Physiol. 173 279.
- Gonda et al. (2008) Synthetic retinoid CD437 induces mitochondriamediated apoptosis in hepatocellular carcinoma cells. Biochem. Biophys.Res.Commun. 370 629.
- Jiang et al. (2007) GADD45A is a mediator of CD437 induced apoptosis in ovarian carcinoma cells. J.Cell.Physiol. 212 771.
- Holmes et al. (2000) Induction of apoptosis in ovarian carcinoma cells by AHPN/CD437 is mediated by retinoic acid receptors. J.Cell.Physiol. 185 61.
- Eyrolles et al. (1994) Retinobenzoic acids. 6. Retinoid antagonists with a heterocyclic ring. J.Med.Chem. 37 1508.
- Alvarez et al. (2009) C3 halogen and C8″ substituents on stilbene arotinoids modulate retinoic acid receptor subtype function. ChemMedChem. 4 1630.
- Busby et al. (2011) Identification of a novel non-retinoid pan inverse agonist of the retinoic acid receptors. ACS Chem.Biol. 6 618.
- Egea et al. (2002) Molecular recognition of agonist ligands by RXRs. Mol.Endocrinol. 16 987.
- Artis et al. (2009) Scaffold-based discovery of indeglitazar, a PPAR panactive anti-diabetic agent. Proc.Natl.Acad.Sci.USA 106 262.
- Mukherjee et al. (1997) Sensitization of diabetic and obese mice to insulin by retinoid X receptor agonists. Nature 386 407.
- Liu et al. (2002) Mechanism of selective retinoid X receptor agonistinduced hypothyroidism in the rat. Endocrinology 143 2880.
- Leibowitz et al. (2006) Biological characterization of a heterodimerselective retinoid X receptor modulator: potential benefits for the treatment of type 2 diabetes. Endocrinology 147 1044.
- Nahoum et al. (2007) Modulators of the structural dynamics of the retinoid X receptor to reveal receptor function. Proc.Natl.Acad.Sci.USA 104 17323.
- Lala et al. (1996) Activation of specific RXR heterodimers by an antagonist of RXR homodimers. Nature 383 450.
- Cesario et al. (2001) The rexinoid LG100754 is a novel RXR:PPARγ agonist and decreases glucose levels in vivo. Mol.Endocrinol. 15 1360.
- Schulman et al. (1997) The phantom ligand effect: allosteric control of transcription by the retinoid X receptor. Genes Dev. 11 299.
- Sato et al. (2010) The “phantom effect” of the rexinoid LG100754: structural and functional insights. PLoS One 5 e15119.
- Ebisawa et al. (1999) Retinoid X receptor-antagonistic diazepinylbenzoic acids. Chem.Pharm.Bull. (Tokyo) 47 1778.
- Umemiya et al. (1997) Action mechanism of retinoid-synergistic dibenzodiazepines. Biochem.Biophys.Res.Commun. 233 121.
- Calleja et al. (2006) Genetic and pharmacological evidence that a retinoic acid cannot be the RXR-activating ligand in mouse epidermis keratinocytes. Genes Dev. 20 1525.
- Kane et al. (2010) Identification of 9-cis-retinoic acid as a pancreas-specific autacoid that attenuates glucose-stimulated insulin secretion. Proc.Natl. Acad.Sci.USA. 107 21884.
- de Urquiza et al. (2000) Docosahexaenoic acid, a ligand for the retinoid X receptor in mouse brain. Science 290 2140.
- LeMotte et al. (1996) Characterization of synthetic retinoids with selectivity for retinoic acid or retinoid X nuclear receptors. Biochim.Biophys.Acta.Gen. Subjects 1289 298.
- Itoh et al. (2008) Structural basis for the activation of PPARγ by oxidized fatty acids. Nat.Struct.Mol.Biol. 15 924.
- Niemoller and Bazan (2009) Docosahexaenoic acid neurolipidomics. Prostaglandins Other Lipid Mediat. 91 85.
- Zomer et al. (2000) Pristanic acid and phytanic acid: naturally occurring ligands for the nuclear receptor peroxisome proliferatoractivated receptor α. J.Lipid.Res. 41 1801.
- Schupp et al. (2007) A widely used retinoic acid receptor antagonist induces peroxisome proliferator-activated receptor-γ activity. Mol.Pharmacol. 71 1251.
- Dawson et al. (2001) Apoptosis induction in cancer cells by a novel analogue of 6-[3-(1-adamantyl)-4-hydroxyphenyl]-2-naphthalenecarboxylic acid lacking retinoid receptor transcriptional activation activity. Cancer Res. 61 4723.
- Chapman et al. (2003) Hydrolysis of 4-HPR to atRA occurs in vivo but is not required for retinamide-induced apoptosis. Arch.Biochem.Biophys. 419 234.
- Cammas et al. (2010) Retinoic acid receptor (RAR)-α is not critically required for mediating retinoic acid effects in the developing mouse retina. Invest.Ophthalmol.Vis.Sci. 51 3281.
- Kim et al. (2007) Regulation of mucin gene expression by CREB via a nonclassical retinoic acid signaling pathway. Mol.Cell.Biol. 27 6933.
- Geissmann et al. (2003) Retinoids regulate survival and antigen presentation by immature dendritic cells. J.Exp.Med. 198 623.
- Stehlin-Gaon et al. (2003) All-trans retinoic acid is a ligand for the orphan nuclear receptor ROR beta. Nat.Struct.Biol. 10 820.
- Shaw et al. (2003) Retinoic acid is a high affinity selective ligand for the peroxisome proliferator-activated receptor β/δ. J.Biol.Chem. 278 41589.
- Zhou et al. (2010)The orphan nuclear receptor TR4 is a vitamin A-activated nuclear receptor. J.Biol.Chem. 286 2877.
- Kruse et al. (2008) Identification of COUP-TFII orphan nuclear receptor as a retinoic acid-activated receptor. PLoS Biol. 6 e227.
- Kang et al. (1999) Mannose 6-phosphate/insulin-like growth factor II receptor mediates the growth-inhibitory effects of retinoids. Cell Growth Differ. 10 591.
- Rajawat et al. (2010) Autophagy: a target for retinoic acids. Autophagy 6 1224.
- Poon and Chen (2008) Retinoic acid-gated sequence-specific translational control by RARα. Proc.Natl.Acad.Sci.USA 105 20303.
- Maghsoodi et al. (2008) Retinoic acid regulates RARα-mediated control of translation in dendritic RNA granules during homeostatic synaptic plasticity. Proc.Natl.Acad.Sci.USA 105 16015.
- Aoto et al. (2008) Synaptic signaling by all-trans retinoic acid in homeostatic synaptic plasticity. Neuron 60 308.
- Chen and Napoli (2008) All-trans-retinoic acid stimulates translation and induces spine formation in hippocampal neurons through a membraneassociated RARα. FASEB J. 22 236.
- Duester (2000) Families of retinoid dehydrogenases regulating vitamin A function: production of visual pigment and retinoic acid. Eur. J.Biochem. 267 4315.
- Haeseleer et al. (2002) Dual-substrate specificity short chain retinol dehydrogenases from the vertebrate retina. J.Biol.Chem. 277 45537.
- Duester et al. (2003) Cytosolic retinoid dehydrogenases govern ubiquitous metabolism of retinol to retinaldehyde followed by tissue-specific metabolism to retinoic acid. Chem.Biol.Interact. 143 201.
- Bhat et al. (1995) Cloning of a cDNA encoding rat aldehyde dehydrogenase with high activity for retinal oxidation. Gene 166 303.
- Penzes et al. (1997) Cloning of a rat cDNA encoding retinal dehydrogenase isozyme type I and its expression in E. coli. Gene 191 167.
- Wang et al. (1996) Cloning of a cDNA encoding an aldehyde dehydrogenase and its expression in Escherichia coli. Recognition of retinal as substrate. J.Biol.Chem. 271 16288.
- Zhao et al. (1996) Molecular identification of a major retinoic-acidsynthesizing enzyme, a retinaldehyde-specific dehydrogenase. Eur.J.Biochem. 240 15.
- Mic et al. (2000) RALDH3, a retinaldehyde dehydrogenase that generates retinoic acid, is expressed in the ventral retina, otic vesicle and olfactory pit during mouse development. Mech.Dev. 97 227.
- Lin et al. (2003) Mouse retinal dehydrogenase 4 (RALDH4), molecular cloning, cellular expression, and activity in 9-cis-retinoic acid biosynthesis in intact cells. J.Biol.Chem. 278 9856.
- Fujii et al. (1997) Metabolic inactivation of retinoic acid by a novel P450 differentially expressed in developing mouse embryos. EMBO J. 16 4163.
- White et al. (1997) cDNA cloning of human retinoic acid-metabolizing enzyme (hP450RAI) identifies a novel family of cytochromes P450. J.Biol.Chem. 272 18538.
- White et al. (2000) Identification of the human cytochrome P450, P450RAI-2, which is predominantly expressed in the adult cerebellum and is responsible for all-trans-retinoic acid metabolism. Proc.Natl. Acad.Sci.USA 97 6403.
- Taimi et al. (2004) A novel human cytochrome P450, CYP26C1, involved in metabolism of 9-cis and all-trans isomers of retinoic acid. J.Biol.Chem. 279 77.
- Soprano and Blaner (1994) Plasma retinol-binding protein. In: The Retinoids: Biology, Chemistry, and Medicine. New York. Raven Press 257.
- Kanai et al. (1968) Retinol-binding protein: the transport protein for vitamin A in human plasma. J.Clin.Invest. 47 2025.
- Benson and Dwulet (1983) Prealbumin and retinol binding protein serum concentrations in the Indiana type hereditary amyloidosis. Arthritis Rheum. 26 1493.
- Isken et al. (2008) RBP4 disrupts vitamin A uptake homeostasis in a STRA6-deficient animal model for Matthew-Wood syndrome. Cell Metab. 7 258.
- Blaner (2007) STRA6, a cell-surface receptor for retinol-binding protein: The plot thickens. Cell Metab. 5 164.
- Kawaguchi et al. (2007) A membrane receptor for retinol binding protein mediates cellular uptake of vitamin A. Science 315 820.
- Ong and Chytil (1975) Specificity of cellular retinol-binding protein for compounds with vitamin A activity. Nature 255 74.
- Delva et al. (1999) Physical and functional interactions between cellular retinoic acid binding protein II and the retinoic acid-dependent nuclear complex. Mol.Cell.Biol. 19 7158.
- Budhu and Noy (2002) Direct channeling of retinoic acid between cellular retinoic acid-binding protein II and retinoic acid receptor sensitizes mammary carcinoma cells to retinoic acid-induced growth arrest. Mol.Cell.Biol. 22 2632.
- Schug et al. (2007) Opposing effects of retinoic acid on cell growth result from alternate activation of two different nuclear receptors. Cell 129 723.
- Formelli et al. (1989) Plasma retinol level reduction by the synthetic retinoid fenretinide: a one year follow-up study of breast cancer patients. Cancer Res. 49 6149.
- Bourguet et al. (1995) Crystal structure of the ligand-binding domain of the human nuclear receptor RXR-α. Nature 375 377.
- Renaud et al. (1995) Crystal structure of the RAR-γ ligand-binding domain bound to all-trans retinoic acid. Nature 378 681.
- Rastinejad et al. (2000) Structure of the RXR-RAR DNA-binding complex on the retinoic acid response element DR1. EMBO J. 19 1045.
- Chandra et al. (2008) Structure of the intact PPAR-γ-RXR- nuclear receptor complex on DNA. Nature 456 350.
About Tocris Bioscience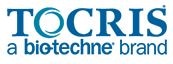
Tocris Bioscience is your trusted supplier of high-performance life science reagents, including receptor agonists & antagonists, enzyme inhibitors, ion channel modulators, fluorescent probes & dyes, and compound libraries. Our catalog consists of over 4,500 research tools, covering over 400 protein targets enabling you to investigate and modulate the activity of numerous signaling pathways and physiological processes.
We have been working with scientists for over 30 years to provide the life science community with research standards, as well as novel and innovative research tools. We understand the need for researchers to trust their research reagents, which is why we are committed to supplying our customers with the highest quality products available, so you can publish with confidence.
Tocris is part of the protein sciences division of Bio-Techne, which also includes the best in class brands R&D Systems, Novus Biologicals, ProteinSimple, and Advanced Cell Diagnostics. Bio-Techne has united these brands to provide researchers with a full portfolio of research reagents, assays, and protein platforms. For more information on Bio-Techne and its brands, please visit bio-techne.com.
Sponsored Content Policy: News-Medical.net publishes articles and related content that may be derived from sources where we have existing commercial relationships, provided such content adds value to the core editorial ethos of News-Medical.Net which is to educate and inform site visitors interested in medical research, science, medical devices, and treatments.